Chapter III. Re-establishing Tolerance
Rev Diabet Stud,
2012,
9(4):305-318 |
DOI 10.1900/RDS.2012.9.305 |
Targeted Antigen Delivery to DEC-205+ Dendritic Cells for Tolerogenic Vaccination
Cathleen Petzold1, Sonja Schallenberg2, Joel N.H. Stern3, Karsten Kretschmer2
1Immunotolerance in Regeneration, Center for Regenerative Therapies Dresden, Dresden, Germany
2Immunotolerance in Regeneration, Center for Regenerative Therapies, Dresden, Germany
3Department of Cancer Immunology and AIDS, Harvard Medical School, Dana-Farber Cancer Institute, Boston, MA, USA
Address correspondence to: Karsten Kretschmer, e-mail: karsten.kretschmer@crt-dresden.de
Manuscript submitted December 22, 2012; resubmitted February 1, 2013; accepted February 7, 2013.
Keywords: type 1 diabetes, immune tolerance, dendritic cell, regulatory T cell, DEC-205, Foxp3
Abstract
Dendritic cells (DCs) and Foxp3-expressing CD4+ regulatory T (Treg) cells play non-redundant roles in the maintenance of peripheral tolerance to self-antigens, thereby preventing fatal autoimmunity. A common hallmark of intra- and extra-thymic Treg cell lineage commitment is the induction of Foxp3 expression as a consequence of appropriate T cell receptor engagement with MHC class II:agonist ligand. It has now become increasingly clear that agonist ligand presentation by immature DCs in the steady state induces T cell tolerance by both recessive and dominant mechanisms, rather than promoting productive T helper cell responses. In this context, the ability of steady-state DCs to promote the extrathymic conversion of initially naïve CD4+Foxp3- T cells into Foxp3+ Treg cells is of particular interest as it provides novel perspectives to enhance antigen-specific Treg cell function in clinical settings of unwanted immunity, such as β-cell autoimmunity.
Abbreviations: Ag - antigen; BDCA – blood dendritic cell antigen; BST2 – bone marrow stromal cell Ag 2 (CD317); C57BL/6 – inbred strain C57 black 6; CIRE – C-type lectin immune receptor; Clec9A – C-type lectin domain family 9, member A; CpG – cytosine-phosphate-guanosine; DC – dendritic cell; DCIR2 – DC-inhibitory receptor-2; DC-SIGN – DC-specific ICAM-3-grabbing non-integrin; DEC-205 – dendritic and epithelial cells, 205 kDa (CD205); Dectin-1 – DC-associated C-type lectin-1; DNA – deoxyribonucleic acid; DTR - diphtheria toxin receptor; EAE – experimental autoimmune encephalomyelitis; Fab – fragment antigen-binding; Fc – fragment, crystallizable; Foxp3 – forkhead box P3; Gag – group-specific antigen; GFP – green fluorescent protein; HA - hemagglutinin; hDEC-205 – human DEC-205; HEL – hen egg lysozyme; HIV – human immunodeficiency virus; IFN-γ – interferon gamma; Ig – immunoglobulin; IL – interleukin; i.m. – intramuscular; i.n. – intranasal; i.p. – intraperitoneal; IPEX – immune dysfunction, polyendocrinopathy, enteropathy, X-linked; i.v. – intranvenous; KLH – keyhole limpet hemocyanin; LC – Langerhans cell; LPS – lipopolysaccharide ; mAb – monoclonal antibody; MHC – major histocompatibility complex; MOG - myelin oligodendrocyte glycoprotein; mRNA – messenger ribonucleic acid; NLDC – non-lymphoid dendritic cell; NOD – non-obese diabetic; NY-ESO-1 – New York esophageal squamous cell carcinoma 1; OT-I – ovalbumin-specific T cell, class I MHC-restricted; OVA – ovalbumin; pDC – plasmacytoid DC; PLP – proteolipid protein; poly(I:C) - polyinosinic:polycytidylic acid; Rag1 – recombination activating gene-1;RIP – rat insulin promoter; s.c. – subcutaneous; scFv – single-chain variable fragment; Siglec-H – sialic acid binding immunoglobulin-like lectin H; SJL – Swiss/Jackson Laboratory (mouse strain); T1D – type 1 diabetes; TAP – transporter associated with Ag processing; TCR – T cell receptor; TGF-β – transforming growth factor beta; Th – T helper; TLR – toll-like receptor; Treg – T regulatory
1. Introduction
CD4+CD25+ regulatory T (Treg) cells expressing the forkhead box transcription factor Foxp3 have been implicated in both the breakdown of self-tolerance and the restoration of immune homeostasis in type diabetes (T1D). As an example, T1D represents a major component of the IPEX (immune dysfunction, polyendocrinopathy, enteropathy, X-linked) syndrome [1-3] that affects patients with abrogated Treg cell function due to mutations in the FOXP3 gene [4-6]. Studies have been performed in adult non-obese diabetes (NOD) mice with transgenic expression of the β-cell-reactive BDC2.5 T cell receptor (TCR) on CD4+ T cells and the human diphtheria toxin receptor (DTR) selectively in Foxp3+ Treg cells. These studies provided a striking example of the ability of Foxp3+ Treg cells to restrain destructive β-cell autoimmunity. While it is well known that the BDC2.5 TCR efficiently prevents the development of spontaneous autoimmune diabetes [7], acute Foxp3+ Treg cell ablation triggers autoimmune β-cell destruction within 8 days after diphtheria toxin administration, in both female and male NOD.BDC2.5 × Foxp3DTR mice [8].
In contrast, Foxp3+ Treg cells have attracted considerable attention as promising gain-of-function targets to achieve tolerance in clinical settings of unwanted immunity (such as β-cell autoimmunity), without compromising protective immune responses to malignant and infectious insults. The ability of β-cell-reactive Foxp3+ Treg cells to prevent or even reverse spontaneously developing diabetes in NOD mice has been demonstrated in early studies, employing in vivo administration of naturally occurring BDC2.5+ Treg cells that had been expanded ex vivo [9, 10], and BDC2.5+ Treg cells that had been artificially generated in vitro either by ectopic expression of Foxp3 [11] or transforming growth factor β (TGF-β)-mediated induction of Foxp3 expression [12]. Conceptually, the in vivo application of in vitro expanded Treg cells is inevitably limited to antigen (Ag) specificities preformed in vivo. In contrast, the conversion of initially naïve CD4+Foxp3- T cells offers the possibility of generating Foxp3+ Treg cells with any desired Ag specificity. Here, we provide an overview on accumulating evidence that the extrathymic conversion of initially non-regulatory CD4+Foxp3- T cells into Foxp3+ Treg cells, by targeted delivery of self-Ag to tissue-residing DEC-205+ dendritic cells (DCs), may represent a suitable approach to deal with organ-specific autoimmunity.
2. Dendritic cells in immune tolerance
In mice, the following main DC subsets can be distinguished based on their migratory behavior and differential expression of surface markers:
1. The population of lymphoid-resident DCs, which samples local Ags for presentation to neighboring T cells in spleen and lymph nodes, including (i) conventional CD11c+ DCs, which can be further subdivided into CD8+CD11blow and CD8-CD11bhigh DCs, and (ii) CD11clowCD11b-B220high plasmacytoid DCs.
2. Migratory DCs, which include epidermal CD11clowCD8-CD11bhigh Langerhans cells (LCs) as well as dermal CD103+ DCs and CD11b+ DCs, travel from peripheral tissues to transport tissue Ags to draining lymph nodes.
Additionally, these DC subsets differentially express several lectin surface receptors. While the expression of DCIR2 and Siglec-H appears largely exclusive to conventional CD11c+CD8- DCs and plasmacytoid DCs, respectively, DEC-205 and Langerin are co-expressed on conventional CD11c+CD8+ DCs, LCs, and migratory DCs.
Currently, it appears to be generally accepted that DCs in peripheral lymphoid tissues control the induction of adaptive immune responses against pathogens, and play an indispensable role in maintaining self-tolerance. This notion is probably best exemplified by the breakdown of T cell tolerance and spontaneous development of fatal autoimmunity in mice with diphtheria toxin A expression selectively in CD11c+ DCs, resulting in the constitutive ablation of conventional DCs, plasmacytoid DCs, and LCs [13]. In contrast, mice with constitutive ablation of only conventional DCs (but not plasmacytoid DCs and Langerhans cells) remained healthy, and lacked obvious signs of autoimmunity [14], suggesting that peripheral DC subsets exert specialized functions in maintaining peripheral tolerance. To protect against fatal autoimmunity, steady-state DCs employ various mechanisms, including the production of soluble factors with known immunoregulatory function (such as interleukin 10 (IL-10) or TGF-β) [15-18], and the induction of both recessive and dominant tolerance in peripheral T cells (see below).
With regard to DC-based immunotherapeutic strategies to induce Ag-specific T cell tolerance in vivo, DCs loaded with Ags under tolerogenic culture conditions have shown efficacy in various preclinical settings of unwanted immunity [19-22], including autoimmune diabetes [23-25]. While these approaches require reinfusion of in vitro manipulated DCs [26], Ag presentation by tolerogenic DCs in their physiological milieu can be achieved through in vivo Ag delivery to lectin surface receptors (such as DEC-205) that serve as Ag uptake and processing receptors for DCs.
3. Targeted Ag delivery to DEC-205+ DCs in vivo
DEC-205 (CD205) is a type I transmembrane surface protein that belongs to the macrophage mannose receptor family of C-type lectin endocytic receptors, which also includes the macrophage mannose receptor and the phospholipase A2 receptor [27]. Structurally, this family is characterized by an extracellular domain, comprised of a cysteine-rich N-terminal domain, a fibronectin type II domain and multiple C-type lectin-like domains, which is followed by a single transmembrane domain and a short cytoplasmic tail. While the natural ligand of DEC-205 remains to be determined, studies employing the rat monoclonal antibody (mAb) NLDC 145 [28] as surrogate ligand to murine DEC-205 revealed that the cytoplasmic DEC-205 tail mediates ligand uptake by receptor-mediated endocytosis and transportation of endocytosed anti-DEC-205 mAb:DEC-205 complexes to late endosomes/MHC class II (MHC-II) compartments [27], resulting in MHC-II Ag presentation to CD4+ T cells (Figure 1). Moreover, anti DEC-205 mAb:DEC-205 receptor complexes can enter the 'exogenous' MHC class I (MHC-I) pathway in a transporter associated with Ag processing (TAP)-dependent manner, leading to MHC-I Ag cross-presentation to CD8+ T cells [29-32] (Figure 1).
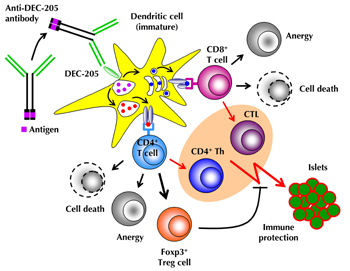 |
 |
Figure 1. Mechanisms of Ag-specific induction of peripheral T cell tolerance through DEC-205+ DCs. Agonist ligands (antigenic peptide or whole protein), fused to the C-terminus of the IgH chain of recombinant antibodies, directed against the endocytic receptor DEC-205 (CD205), are selectively delivered to DEC-205+ DCs. Upon binding of anti-DEC-205 fusion antibodies to DEC-205, which is highly expressed on DCs residing in T cell areas of peripheral lymphoid tissues, mAb:receptor complexes are internalized and targeted to late endosomes/MHC-II compartments. Processed proteins can enter either the 'exogenous' MHC class I pathway, leading to Ag cross-presentation to CD8+ T cells, or the classical MHC class II pathway, resulting in Ag presentation to CD4+ T cells. Upon high-dose Ag stimulation by immature DEC-205+ DCs (i.e. administration of ≥1 μg anti-DEC-205 mAb fused to antigenic peptide), Ag-specific CD4+ and CD8+ T cells are physically eliminated (cell death) or acquire an immunologically unresponsive state (anergy). Low-dose Ag stimulation by immature DCs (≤ 0.1 μg fusion mAb) favors the conversion of initially naïve CD4+CD25-Foxp3- T cells into stable Foxp3+ Treg cells, rather than the induction of apoptosis or anergy. DEC-205+ DC targeting in conjunction with the administration of DC maturation stimuli (e.g. agonistic anti-CD40 mAbs, poly(I:C), CpG, lipopolysaccharide (LPS), or toll-like receptor 7/8 (TLR7/8) agonists) represents an effective means for inducing strong T cell responses by CD4+ T helper (Th) and CD8+ cytotoxic T cells (CTL) (highlighted in pink). |
|
Upon high-dose administration in vivo, fluorochrome-conjugated anti-DEC-205 mAbs are rapidly captured and efficiently taken up by the majority of lymphoid-resident conventional CD8+ (but not CD8-) DCs, as well as migratory CD103+ DCs and LCs [33]. However, no appreciable anti-DEC-205 mAb binding was observed to CD19-CD11c+ splenocytes or CD19+ B cells that exhibit low surface expression of DEC-205 [34]. For the selective delivery to DEC-205+ DCs, the Ag of interest (either whole protein or Ag peptide) can be conjugated to the natural NLDC-145 mAb by chemical crosslinking. Alternatively, the Ag can be fused to the C-terminus of the IgH chain of recombinant anti-DEC-205 mAbs (Figure 1), whose original rat IgG2a constant regions were replaced with mouse IgG1 constant regions, carrying point mutations that interfere with Fc receptor binding [35]. This mode of Ag delivery has been shown to be several orders of magnitude more efficient than free peptide administration in mediating MHC-I (≥1000-fold) and MHC-II (≥100-fold) presentation [29, 36]. Importantly, depending on the DC maturation stage, TCR stimulation through Ag recognition on tissue-residing DEC-205+ DCs can promote either the differentiation of Ag-specific T effector cells or immunological T cell tolerance.
4. DEC-205+ DC targeting to enhance Ag-specific T cell immunity
In the steady state (i.e. in the absence of infectious agents or deliberate DC maturation stimuli), DCs exhibit a largely immature phenotype. Binding of the anti-DEC-205 mAb alone is insufficient to promote maturation and activation of DEC-205+ DCs, as exemplified by unaltered low expression levels of T cell costimulatory molecules such as CD40, CD80, and CD86 [34, 37]. However, anti-DEC-205-targeted Ag delivery, in conjunction with co-administration of adjuvant (DC maturation stimuli such as agonistic anti-CD40 mAbs, poly(I:C), cytosine-phosphate-guanine (CpG), lipo-polysaccharide (LPS), or toll-like receptor 7/8 (TLR7/8) agonists), results in enhanced Ag presentation capacity and increased expression of T cell costimulatory molecules by maturing DEC-205+ DCs [38-40]. This approach represents an effective means to elicit robust Ag-specific immunity characterized by high frequencies of IL-2-/IFN-γ-producing CD4+ T helper and CD8+ cytotoxic T cells, production of high-affinity IgG antibodies, and long-lived T cell memory [36, 41, 42]. Combined DEC-205+ DC targeting and deliberate DC maturation has been demonstrated to elicit productive immune responses against pathogens, including viruses [36, 43-45], bacteria [39, 46, 47], and parasites [38, 48], but also against tumor Ags [40, 49-51].
In humans, the expression pattern of DEC-205 (hDEC-205) on tissue-residing DCs is poorly defined, but hDEC-205 expression can be detected on a small proportion of immature DCs from peripheral blood, and is further upregulated upon DC maturation in vitro [52]. With regard to the major DC populations in peripheral blood, hDEC-205 is expressed at high levels on mature CD11c+CD123-BDCA-3+ myeloid DCs and at low levels on CD11c-CD123+BDCA-2+BDCA-4+ plasmacytoid DCs [53, 54]. This observations appear consistent with co-expression of hDEC-205 and CD11c on DCs residing in the T cell areas of human spleen and lymph nodes [55]. Compared to the mouse homolog, hDEC-205 protein exhibits ~80% identity, suggesting functional properties that are conserved between species. In fact, humanized recombinant anti-hDEC-205 mAbs fused to HIV-derived Gag peptides efficiently target DCs and elicit strong CD4+ and CD8+ T cell responses in vivo, both in mice with CD11c promoter-driven transgenic expression of hDEC-205 [56] and in rhesus macaques [57]. Finally, it appears worthwhile mentioning that a fully human anti-hDEC-205 mAb linked to NY-ESO-1 is currently in phase 1-2 development (Celldex Therapeutics, USA) for the treatment of a variety of cancers that express the tumor Ag NY-ESO-1.
5. DEC-205+ DC targeting to promote Ag-specific T cell tolerance
5.1 Recessive tolerance
Hawiger et al. first demonstrated that Ag presentation by steady-state DCs can result in the induction of immunological unresponsiveness of Ag-specific CD4+ T cells, rather than productive T helper (Th) cell responses [34] (Figure 1). These experiments involved the adoptive transfer of TCR transgenic CD4+ 3A9 T cells, which are reactive to the model Ag hen egg lysozyme (HEL), and targeted delivery of the respective agonist peptide to DEC 205+ DCs in immunocompetent hosts. Upon local administration of recombinant anti-DEC-205/HEL fusion mAbs (200 ng, s.c. into the footpad), Ag presentation by immature DEC-205+ DCs initially induced Ag-specific T cell activation, as revealed by cell division and production of IL-2 (but not IFN-γ). However, the 3A9 T cells were then rapidly deleted. Residual 3A9 T cells that did not undergo deletion were found to be refractory to antigenic restimulation in vivo [58], which could be attributed to mechanisms that required increased expression of CD5 [58]. In these studies, the expression of Foxp3 in DEC-205+ DC-primed CD4+ T cells was not analyzed. Importantly, the observation of DEC-205+ DC-targeted induction of Ag-specific CD4+ T cell deletion and anergy could subsequently be extended to Ag-specific CD8+ T cells (Figure 1), employing TCR transgenic CD8+ OT-I T cells reactive to ovalbumin and DEC-205+ DC targeting of whole ovalbumin protein [29]. It is also worthwhile mentioning that DEC-205+ DC-targeted induction of recessive CD4+ and CD8+ T cell tolerance appears effective over a relatively broad range of anti-DEC-205 mAb doses (0.2-15 μg), but is consistently abrogated by co-administration of DC maturation stimuli such as agonistic anti-CD40 mAbs [29, 34, 42].
5.2 Dominant tolerance
In addition to the induction of recessive tolerance, the ability of steady-state DEC-205+ DCs to promote dominant tolerance by promoting the extrathymic de novo generation of Foxp3+ Treg cells (Figure 1) has now been conclusively demonstrated in several independent studies. Before Foxp3 fluorochrome reporter mice became commonly available, initial studies employed Foxp3 mAbs for the analysis of Foxp3 expression in single cells and influenza hemagglutinin (HA)-reactive TCR transgenic (TCR-HA) CD4+ T cells that have a naïve Foxp3-CD25- phenotype, and that had been isolated from Rag-/- mice and injected into immunocompetent recipients [37, 59]. In this adoptive transfer model, careful titration experiments demonstrated that a single dose injection of minute amounts of recombinant anti-DEC-205/HA fusion mAb (~50 ng, corresponding to ~5 ng Ag) is necessary and sufficient to extrathymically convert up to 30% of initially CD4+Foxp3-CD25- T cells into functional CD25+Foxp3+ Treg cells, and revealed an inverse relationship of cell division and Foxp3 upregulation. Thus, conditions that limited proliferation of converting T cells (i.e. low Ag dose, immature DC maturation stage, reduced IL-2R, and enhanced TGF-β receptor (TGF-βR) signaling) increased the efficiency of Foxp3 upregulation [37], probably through opposing cell cycle-dependent epigenetic silencing of the gene locus encoding Foxp3 [60].
More recently, the concept of extrathymic Foxp3+ Treg cell induction by low-dose DEC-205+ DC targeting has been further corroborated by studies employing naive CD4+Foxp3GFP- T cells from peripheral lymphoid tissues of Foxp3 fluorochrome reporter mice on a variety of different genetic backgrounds. This includes TCR transgenic T cells reactive to non-self-Ags (HA [61], ovalbumin [17, 61], and HY [62]), and to self-Ags (chromogranin A/BDC2.5 mimotope [63] and unpublished observation, insulin B chain [64], and myelin oligodendrocyte glycoprotein [33]). However, with regard to Ag-specific proliferative expansion of preformed Foxp3+ Treg cell populations in the steady state, administration of anti-DEC-205 mAbs appears rather ineffective in inducing cell division in Foxp3+ Treg cells, once they have been generated by DEC-205+ DC targeting [17, 62].
6. Extrathymic Foxp3+ Treg cell induction in nonmanipulated mice
Recently, we addressed the question of whether DEC-205+ DCs promote peripheral Foxp3+ Treg cell induction not only from TCR transgenic T cells upon targeted Ag delivery, but also from non-TCR transgenic T cells in nonmanipulated mice. Initially, we analyzed early events during extrathymic Treg cell induction from TCR transgenic CD4+Foxp3- T cells by low-dose DEC-205+ DC targeting because of the lack of definitive markers to discriminate Foxp3+ Treg cells that have been induced either intra- or extra-thymically [61]. We identified a panel of surface molecules on DC-primed CD4+Foxp3- T cells whose differential regulation marks distinct stages during their differentiation into Foxp3+ Treg cells (Figure 2). Shortly after TCR stimulation by cognate Ag presentation on DEC-205+ DCs, Foxp3- T cells with a naïve phenotype (CD62LhighCD69-CD25-) concomitantly downregulate CD62L and upregulate CD69 expression (Figure 2A). At this stage, converting CD62LintCD69+ T cells are still CD25-Foxp3- (Figure 2B), but many are already poised to upregulate CD25 and Foxp3 expression (Figure 2C). Developmental progression of such CD25-Foxp3- Treg cell precursors in vivo is characterized by the acquisition of CD25 expression, with CD25+Foxp3- T cells representing the penultimate stage towards differentiation into Foxp3+ Treg cells. In fact, the majority of CD25+Foxp3- Treg cell precursors is precommitted to undergo IL-2-driven upregulation of Foxp3 expression, a process that does not require continued TCR or TGF-βR stimulation (Figure 2B, C). The sequence of events during DEC-205+ DC-targeted Foxp3+ Treg cell induction is schematically depicted in Figure 3.
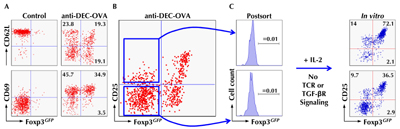 |
 |
Figure 2. Tracking extrathymic differentiation of Agspecific CD4+ T cells during DEC-205+ DCmediated Foxp3 induction. Fluorescence-activated cell sorting (FACS)-purified populations of naïve CD4+ T cells (CD62LhighCD25-Foxp3GFP-) with transgenic expression of an ovalbumin (OVA)-reactive T cell receptor (TCR) (DO11.10) were adoptively transferred into congenic, immunocompetent mice. The next day, recipients were either left untreated (control) or injected with 250 ng recombinant anti-DEC-205 antibodies fused to whole OVA protein. At day 5, congenic marker+ DO11.10 T cells were tracked in lymph nodes of recipient mice by flow cytometry. A, B: Differential expression of CD62L and CD69 (A) and CD25 (B) during anti-DEC-205/OVA-mediated induction of Foxp3GFP expression. C: Populations of CD25- and CD25+ cells among Foxp3GFP-DO11.10+ T cells were purified by flow cytometry, as indicated by the lines with an arrowhead (left). At day 3 of IL-2-supplemented cultures, in the absence of deliberate TCR and transforming growth factor β receptor (TGF-βR) stimulation, Foxp3GFP and CD25 expression was determined by flow cytometry (right). Adapted from Schallenberg et al., 2010 [61]. |
|
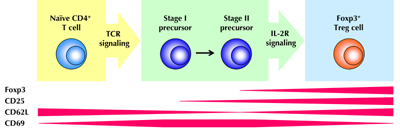 |
 |
Figure 3. Extrathymic differentiation of CD4+Foxp3- T cells into Foxp3+ Treg cells. Upon TCR stimulation through recognition of cognate Ag presented by peripheral lymphoid tissue-residing, immature DEC-205+ DCs, naïve CD4+Foxp3+ T cells (CD62LhighCD69-CD25-) undergo a series of differentiation steps that are characterized by differential surface expression of activation markers. Transient downregulation of CD62L and upregulation of CD69 is accompanied by the gradual acquisition of constitutive CD25 expression. Interleukin 2 receptor (IL-2R) signaling has a non-redundant function in promoting the developmental progression of precommitted CD25+Foxp3+ Treg cell precursors with a CD62LintCD69+ surface marker phenotype into functional Foxp3+ Treg cells. |
|
In subsequent studies, we compared the differential surface marker expression during DEC-205+ DC-targeted Treg cell induction from TCR transgenic T cells with polyclonal CD4+ T cell populations in peripheral lymphoid tissues of non-manipulated Foxp3GFP mice. This resulted in the identification of sizable populations of CD25+Foxp3- precursors precommitted to Foxp3+ Treg cells, providing direct evidence for the generation of extrathymic Treg cells from non-TCR transgenic CD4+ T cells under steady-state conditions. Differentiation of such peripheral CD4+CD25+Foxp3- precursors into Foxp3+ Treg cells was dependent on phosphatidylinositol 3-kinase and IL-2R signaling, but proceeded in the absence of other common γ chain cytokines or TGF-βR signaling.
Overall, these observations on peripheral Foxp3- Treg precursor cells in vivo [61], together with the notion that ex vivo CD8+DEC-205+ DCs induce Foxp3 expression in vitro in initially CD4+Foxp3- T cells without added TGF-β [17], make a strong case that extrathymic Foxp3+ Treg cell development in nonmanipulated mice is mediated by lymphoid tissue-residing CD8+DEC-205+ DCs. The identification of Foxp3- Treg precursor cells provides an opportunity to study the molecular mechanisms of extrathymic Foxp3+ Treg cell generation under physiological conditions (i.e. immunocompetent mice, non-TCR transgenic T cells, non-deliberate T cell stimulation, and physiological cytokine milieu). Such studies may help to develop strategies that further enhance the efficacy of current approaches to promote Ag-specific T cell tolerance in autoimmunity (i.e. combination therapy, see below).
7. Stability and function of DEC-205+ DC-targeted Foxp3+ Treg cells
Current issues in Treg cell biology that are particularly relevant for the development of novel cell-based therapies, designed to deal with unwanted immunity, include the phenotypic and functional stability of Foxp3+ Treg cells. In fact, opinions differ on whether naturally induced Treg cells exhibit a largely stable Foxp3+ phenotype [65], or could be reprogrammed to differentiate into non-regulatory Foxp3- T effector cells in vivo [66-70]. Artificially generated Treg cells with a Foxp3+ suppressor phenotype, which has been elicited in initially naïve Foxp3- T cells through TCR and TGF-βR stimulation in vitro, rapidly downregulate induced Foxp3 expression and lose their suppressor function, both upon restimulation in vitro [71, 72] and reinfusion into immunocompetent mice [73]. Importantly, Foxp3+ Treg cell induction by low-dose DEC-205+ DC targeting in vivo leads to efficient DNA demethylation of conserved CpG motifs within non-coding regions of the Foxp3 gene, a predictive molecular marker for the long-term stability of induced Foxp3 expression [72]. Consequently, the overwhelming majority of Foxp3+ Treg cells, generated in this manner, maintains high levels of Foxp3 expression upon proliferative expansion in vitro [72]. In vivo, DEC-205 DC-targeted Treg cells survive for several months in the absence of the inducing Ag [63, 72], and maintain a stable Foxp3+ suppressor phenotype under inflammatory and autoimmune conditions [37, 63].
The capacity of ex vivo DEC-205+ DC-targeted Foxp3+ Treg cells to suppress the activation of conventional CD4+ T cells was directly demonstrated by the abrogation of T responder cell proliferation in standard co-culture assays [37]. Under inflammatory in vivo conditions, DEC 205+ DC-targeted Foxp3+ Treg cells markedly reduced the accumulation of Ag-specific T effector cells in draining lymph nodes, with residual T effector cells exhibiting abrogated expression of activation markers and production of effector cytokines [37]. While their exact mode of action remains unclear, recent global gene expression analysis revealed that DEC-205+ DC-targeted Foxp3+ Treg cells exhibited a unique mRNA Treg cell signature, as compared to a variety of Foxp3+ Treg cell subsets, either purified from different anatomical locations or artificially generated by different means [74]. These differences included overrepresentation of mRNAs of the canonical Treg cell signature encoding functionally relevant molecules, such as chemokine receptors guiding the homing to different anatomical compartments, and effector molecules involved in Foxp3+ Treg cell-mediated suppressor function, such as CTLA-4 and IL10 [74].
8. Tolerogenic DEC-205+ DC vaccination in autoimmunity
8.1 Autoimmune diabetes
Early studies concerned with the tolerogenic potential of DEC-205+ DC targeting in T1D employed a double-transgenic model of spontaneous autoimmune diabetes [75], consisting of mice that co-express the HA protein as a neo-self Ag in pancreatic β-cells under control of the rat insulin promoter (RIP-HA) and TCR-HA-expressing CD4+ T cells [76]. In the TCR HA × RIP-HA model, repeated injection of newborn mice with the anti DEC 205 mAb NLDC-145, chemically conjugated to synthetic HA peptide, protected the majority of the treated mice from disease development, whereas ~40% of untreated mice progressed towards autoimmune diabetes [75]. Apart from obvious limitations of the TCR-HA × RIP-HA model [77], including unphysiological frequencies of pathogenic CD4+ T cells and restriction of β-cell pathogenicity to a single neo-self-Ag, this study provided the first evidence that the selective delivery of β-cell Ag to steady-state DEC-205+ DCs may represent a feasible approach to Ag-specifically interfere with the development of autoimmune diabetes. However, in this study, the relative contribution of recessive (anergy/deletion of pathogenic TCR-HA+ T cells) and dominant (de novo generation/proliferative expansion of preformed TCR-HA+Foxp3+ Treg cells) tolerance mechanisms to the autoimmune protection of β-cells remains unclear.
Under more physiological conditions of limited frequencies of β-cell-reactive T cells, low numbers of highly diabetogenic CD4+BDC2.5+ T cells with a naïve CD62LhighCD25-Foxp3- phenotype, which had been adoptively transferred into immunocompetent NOD mice, underwent efficient conversion into long-lived Foxp3+BDC2.5+ Treg cells upon targeted delivery of agonistic mimotope peptide to DEC-205+ DCs in recipient mice [63]. Despite induction of a Foxp3+ Treg cell phenotype in up to 35% of initially Foxp3-BDC2.5+ T cells, DEC-205+ DC targeting of the BDC2.5 mimotope did not appreciably interfere with the development of autoimmune diabetes in NOD recipients. Notably, systemic administration of free synthetic BDC2.5 mimotope peptide appeared to accelerate diabetes progression, as compared to equivalent amounts of mimotope peptide fused to recombinant anti-DEC-205 or isotype control Abs which had been administered following the same treatment regimen.
Consistent with the concept that proinsulin is a major self-Ag and primary target during the early stages of T1D development [78-80], low-dose-delivery of whole proinsulin to DEC-205+ DCs in prediabetic NOD mice interfered with the progression towards overt diabetes; 50% of mice that received 4 consecutive injections of 1 μg anti-DEC-proinsulin mAbs at 2 months of age maintained normoglycemia until the end of the observation period at 6 month of age [63]. In contrast, 90% of NOD mice injected with equivalent amounts of isotype control Ab, or left untreated, had progressed towards overt autoimmune diabetes. Evidence for an involvement of Ag-specific Foxp3+ Treg cells in reduced disease incidence includes the observation that co-transfer of spleen cells from anti DEC 205/ proinsulin-treated NOD mice delayed diabetes development in a NOD.Rag1-/- adoptive transfer model [63]. Mechanistically, the higher complexity of whole proinsulin regarding the number of involved MHC-I and MHC-II T cell epitopes, compared with the MHC-II-restricted mimotope peptide, most likely contributes to the increased efficacy of DEC-205+ DC-targeted proinsulin in ameliorating autoimmune diabetes progression. Indeed, β-cell Ag presentation by steady-state DEC-205+ DCs in peripheral lymphoid tissues of NOD mice can result in deletional tolerance of diabetogenic CD8+ T cells (Figure 1), as formally demonstrated in studies employing adoptive transfer of TCR transgenic, β-cell-reactive CD8+ T cells and DEC-205+ DC targeting of the respective mimotope peptide [81, 82]. Approaches to further increase the efficacy of tolerogenic DEC 205+ DC vaccination may include the design of natural β-cell Ag-derived mimotope peptides that, compared to their physiological counterparts, exhibit improved agonistic activity, and thereby enhanced capacity to promote Ag-specific conversion of diabetogenic CD4+Foxp3- T cells into protective Foxp3+ Treg cells [64, 83].
8.2 Autoimmune encephalomyelitis
Additional evidence that DEC-205+ DC targeting may represent a suitable approach to prevent destructive autoimmunity was provided by studies in mouse models of experimental autoimmune encephalomyelitis (EAE), which recapitulate many aspects of human multiple sclerosis [83]. In C57BL/6 mice, high-dose DEC-205+ DC targeting (15 μg) of a myelin oligodendrocyte glycoprotein (MOG)-derived encephalogenic peptide prevented the induction of EAE by the same peptide in complete Freund’s adjuvant, through Ag-specific deletion and anergy induction in residual MOG-reactive T cells [58]. In a passive EAE model, adoptive transfer of pathogenic proteolipid protein (PLP)-specific CD4+ T cells into SJL mice, and subsequent administration of recombinant anti-DEC-205/PLP139-151 fusion antibodies, substantially delayed disease onset and significantly reduced the mean clinical score [84]. In an active EAE model, DEC-205+ DC targeting of PLP139-151 efficiently ameliorated clinical symptoms of PLP139-151-induced EAE in SJL mice, which involved both recessive (deletion of pathogenic CD4+ Th17 cells) and dominant (enhancement of Foxp3+ Treg cell activity) tolerance mechanisms [84]. The ability of Ag-targeted DEC-205+ DCs to induce Foxp3+ Treg cells from encephalogenic CD4+ T cells and to interfere with disease has been further corroborated in recent studies employing the C57BL/6 model of MOG-induced EAE [33].
Overall, the above studies have proven the relevance of tolerogenic DEC-205+ DC vaccination in two distinct models of organ-specific autoimmunity that fundamentally differ in their autoimmune mechanisms and immune effector cells involved in tissue destruction. It will be important to determine whether these findings can be extended to additional autoimmune diseases, such as rheumatoid arthritis or inflammatory bowel disease.
9. Ag-specific induction of T cell tolerance: a unique property of DEC-205+ DCs?
Apart from the endocytic receptor DEC-205, a variety of additional surface markers with differential expression on DC subsets has been assessed for their ability to promote Ag-specific T cell priming upon targeted Ag delivery (Table 1). For this, mAbs (natural mAbs, Fab fragments, whole recombinant Abs, or single-chain Fv recombinant Ab fragments) have been fused or chemically cross-linked to the Ag of interest and administered via different routes (s.c., i.p., i.v., or i.n.). These studies are aimed mainly to enhance Ag-specific CD4+ and CD8+ T cell responses against cancer and microbial infections by co-administration of adjuvant to promote DC maturation [34, 36, 42, 44, 85-90]. Notably, and in striking contrast to anti-DEC-205+ DC targeting [37], anti-CD36 mAb-mediated DC targeting in vivo elicits profound Ag-specific CD4+ and CD8+ T cell responses, regardless of whether DCs had been deliberately activated by co-administration of anti-CD40 mAbs [91]. Interestingly, some surface molecules (CD40, MHC-II, TLR2, and FcγRII/III) failed to mediate appreciable MHC presentation of targeted Ag and activation of Ag-specific T cells in vivo [89].
Table
1.
Targeted Ag delivery to surface molecules on dendritic cells |
|
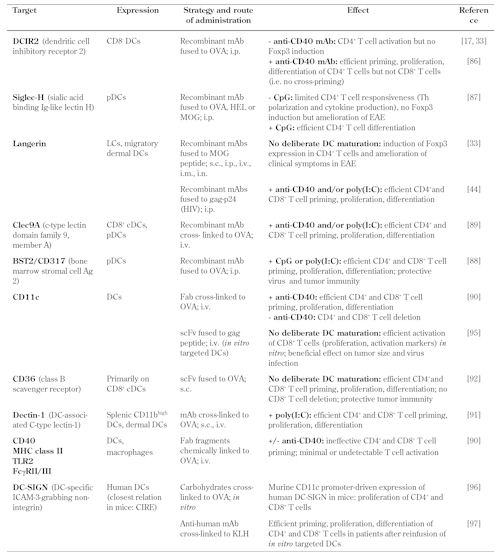 |
 |
Legend:
CIRE – C-type lectin immune receptor, CpG – cytosine-phosphate-guanosine, cDC – conventional dendritic cell, EAE – experimental autoimmune encephalomyelitis, Fab – fragment antigen-binding, HEL – hen egg lysozyme, ICAM-3 - intercellular adhesion molecule 3, i.m. – intramuscular, i.n. – intranasal, i.p. – intraperitoneal, i.v. – intravenous, KLH – keyhole limpet hemocyanin, LC – Langerhans cell, mAb – monoclonal antibody, MHC – major histocompatibility complex, MOG – myelin oligodendrocyte glycoprotein, OVA – ovalbumin, pDC – plasmacytoid dendritic cell, poly(I:C) – polyinosinic:polycytidylic acid, s.c. – subcutaneous, scFv – single-chain variable fragment, Th – T helper, TLR – toll-like receptor. |
|
With regard to promoting Ag-specific T cell tolerance under subimmunogenic conditions (i.e. steady-state DCs, low-dose Ag), anti-Langerin mAb-mediated targeting of Langerin+ DCs in vivo was found to be similarly effective in inducing a Foxp3+ Treg cell phenotype in initially naïve CD4+Foxp3- T cells and in ameliorating clinical symptoms in EAE [33], compared with DEC 205+ DC targeting [84]. Mechanistically, this finding is particularly interesting as the population of Langerin+ DCs, which comprises lymphoid-resident CD8+, migratory CD103+ DCs, and epidermal Langerhans cells [33, 92], co-expresses high levels of DEC-205 [33], suggesting that the same DC subset mediates anti-DEC-205- and anti-Langerin-targeted induction of Foxp3+ Treg cells. In contrast, Ag targeting to DCIR2 on CD8-DCIR2+ conventional DCs [17] or Siglec-H on CD4+Siglec-H+ plasmacytoid DCs [86], which exhibit low or negligible expression of DEC-205, failed to promote peripheral Foxp3+ Treg cell induction. Interestingly, in the absence of deliberate DC maturation stimuli, DCIR2+ DC targeting of cognate Ag was found to promote the proliferative expansion of preformed Ag-specific Foxp3+ Treg cells, rather than their de novo generation from initially naïve CD4+Foxp3- T cells [17]. Finally, and in contrast to DCIR2+ DC targeting [33], Siglec-H+ DC targeting ameliorated clinical symptoms in the MOG-induced C57BL/6 model of EAE [86], possibly by interference with the Ag-specific proliferation of MOG-reactive CD4+ T cells and their differentiation into pathogenic Th1 and Th17 cells [86]. In autoimmune diabetes, the tolerogenic potential of targeted delivery of β-cell Ag to DC surface receptors other than DEC-205 (e.g. Langerin, DCIR2, or Siglec-H) has not been investigated to date.
10. Conclusions
In T1D, robust data that demonstrate the clinical benefit of stimulating tolerogenic mechanisms by administration of free β-cell Ag are not yet available [94]. In this context, the ability to target minute amounts of β-cell antigen (either peptide or protein) selectively to steady-state DEC-205+ DCs has potential as a safe approach to deal with β-cell autoimmunity. The combination of DEC-205+ DC targeting with other immunomodulatory strategies that do not interfere with Foxp3+ Treg cell generation may help to ameliorate possible adverse effects of increased DC and T cell activation on the efficiency of Ag-specific Foxp3+ Treg cell induction. Rational approaches to such combination therapies may include immunosuppressive drugs, costimulatory blockage, anti-CD3 treatment, and modulation of cytokine receptor signaling.
Disclosure: The authors report no conflict of interests.
Acknowledgments:
This work was supported by the Kompetenznetz Diabetes mellitus (Competence Network for Diabetes mellitus) funded by the Federal Ministry of Education and Research (FKZ 01GI0805-07), by a grant from the BMBF to the German Center for Diabetes Research (DZD e.V., FKZ01GI0924), and by the FZT 111 (DFG, Center for Regenerative Therapies Dresden, Cluster of Excellence).
References
- Moraes-Vasconcelos D, Costa-Carvalho BT, Torgerson TR, Ochs HD. Primary immune deficiency disorders presenting as autoimmune diseases: IPEX and APECED. J Clin Immunol 2008. 28(1):11-19. [DOD] [CrossRef]
- Torgerson TR, Ochs HD. Immune dysregulation, polyendocrinopathy, enteropathy, X-linked: Forkhead box protein 3 mutations and lack of regulatory T cells. J Allergy Clin Immunol 2007. 120(4):744-750. [DOD] [CrossRef]
- Wildin RS, Smyk-Pearson S, Filipovich AH. Clinical and molecular features of the immunodysregulation, polyendocrinopathy, enteropathy, X linked (IPEX) syndrome. J Med Genet 2002. 39(8):537-545. [DOD] [CrossRef]
- Chatila TA, Blaeser F, Ho N, Lederman HM, Voulgaropoulos C, Helms C, Bowcock AM. JM2, encoding a fork head-related protein, is mutated in X-linked autoimmunity-allergic disregulation syndrome. J Clin Invest 2000. 106(12):R75-R81. [DOD] [CrossRef]
- Bennett CL, Christie J, Ramsdell F, Brunkow ME, Ferguson PJ, Whitesell L, Kelly TE, Saulsbury FT, Chance PF, Ochs HD. The immune dysregulation, polyendocrinopathy, enteropathy, X-linked syndrome (IPEX) is caused by mutations of FOXP3. Nat Genet 2001. 27(1):20-21. [DOD] [CrossRef]
- Wildin RS, Ramsdell F, Peake J, Faravelli F, Casanova JL, Buist N, Levy-Lahad E, Mazzella M, Goulet O, Perroni L, et al. X-linked neonatal diabetes mellitus, enteropathy and endocrinopathy syndrome is the human equivalent of mouse scurfy. Nat Genet 2001. 27(1):18-20. [DOD] [CrossRef]
- You S, Slehoffer G, Barriot S, Bach JF, Chatenoud L. Unique role of CD4+CD62L+ regulatory T cells in the control of autoimmune diabetes in T cell receptor transgenic mice. Proc Natl Acad Sci USA 2004. 101(Suppl 2):14580-14585. [DOD] [CrossRef]
- Petzold C, Riewaldt J, Watts D, Sparwasser T, Schallenberg S, Kretschmer K. Foxp3+ regulatory T cells in mouse models of type 1 diabetes. Exp Diabetes Res 2013. In press. [DOD]
- Tarbell KV, Petit L, Zuo X, Toy P, Luo X, Mqadmi A, Yang H, Suthanthiran M, Mojsov S, Steinman RM. Dendritic cell-expanded, islet-specific CD4+ CD25+ CD62L+ regulatory T cells restore normoglycemia in diabetic NOD mice. J Exp Med 2007. 204(1):191-201. [DOD] [CrossRef]
- Tarbell KV, Yamazaki S, Olson K, Toy P, Steinman RM. CD25+ CD4+ T cells, expanded with dendritic cells presenting a single autoantigenic peptide, suppress autoimmune diabetes. J Exp Med 2004. 199(11):1467-1477. [DOD] [CrossRef]
- Jaeckel E, von Boehmer H, Manns MP. Antigen-specific FoxP3-transduced T-cells can control established type 1 diabetes. Diabetes 2005. 54(2):306-310. [DOD] [CrossRef]
- Luo X, Tarbell KV, Yang H, Pothoven K, Bailey SL, Ding R, Steinman RM, Suthanthiran M. Dendritic cells with TGF-beta1 differentiate naive CD4+CD25- T cells into islet-protective Foxp3+ regulatory T cells. Proc Natl Acad Sci USA 2007. 104(8):2821-2826. [DOD] [CrossRef]
- Ohnmacht C, Pullner A, King SB, Drexler I, Meier S, Brocker T, Voehringer D. Constitutive ablation of dendritic cells breaks self-tolerance of CD4 T cells and results in spontaneous fatal autoimmunity. J Exp Med 2009. 206(3):549-559. [DOD] [CrossRef]
- Birnberg T, Bar-On L, Sapoznikov A, Caton ML, Cervantes-Barragan L, Makia D, Krauthgamer R, Brenner O, Ludewig B, Brockschnieder D, et al. Lack of conventional dendritic cells is compatible with normal development and T cell homeostasis, but causes myeloid proliferative syndrome. Immunity 2008. 29(6):986-997. [DOD] [CrossRef]
- Travis MA, Reizis B, Melton AC, Masteller E, Tang Q, Proctor JM, Wang Y, Bernstein X, Huang X, Reichardt LF, et al. Loss of integrin avb8 on dendritic cells causes autoimmunity and colitis in mice. Nature 2007. 449(7160):361-365. [DOD] [CrossRef]
- Coombes JL, Siddiqui KR, Arancibia-Carcamo CV, Hall J, Sun CM, Belkaid Y, Powrie F. A functionally specialized population of mucosal CD103+ DCs induces Foxp3+ regulatory T cells via a TGF-beta and retinoic acid-dependent mechanism. J Exp Med 2007. 204(8):1757-1764. [DOD] [CrossRef]
- Yamazaki S, Dudziak D, Heidkamp GF, Fiorese C, Bonito AJ, Inaba K, Nussenzweig MC, Steinman RM. CD8+CD205+ splenic dendritic cells are specialized to induce Foxp3+ regulatory T cells. J Immunol 2008. 181(10):6923-6933. [DOD]
- Akbari O, DeKruyff RH, Umetsu DT. Pulmonary dendritic cells producing IL-10 mediate tolerance induced by respiratory exposure to antigen. Nat Immunol 2001. 2(8):725-731. [DOD] [CrossRef]
- Giannoukakis N, Bonham CA, Qian S, Chen Z, Peng L, Harnaha J, Li W, Thomson AW, Fung JJ, Robbins PD, et al. Prolongation of cardiac allograft survival using dendritic cells treated with NF-kB decoy oligodeoxyribonucleotides. Mol Ther 2000. 1(5 Pt 1):430-437. [DOD] [CrossRef]
- Menges M, Roessner S, Voigtlaender C, Schindler H, Kukutsch NA, Bogdan C, Erb K, Schuler G, Lutz MB. Repetitive Injections of Dendritic Cells Matured with Tumor Necrosis Factor alpha Induce Antigen-specific Protection of Mice from Autoimmunity. J Exp Med 2002. 195(1):15-22. [DOD] [CrossRef]
- Verginis P, Li HS, Carayanniotis G. Tolerogenic Semimature Dendritic Cells Suppress Experimental Autoimmune Thyroiditis by Activation of Thyroglobulin-Specific CD4+CD25+ T Cells. J Immunol 2005. 174(11):7433-7439. [DOD]
- Lim DS, Kang MS, Jeong JA, Bae YS. Semi-mature DC are immunogenic and not tolerogenic when inoculated at a high dose in collagen-induced arthritis mice. Eur J Immunol 2009. 39(5):1334-1343. [DOD] [CrossRef]
- Feili-Hariri M, Falkner DH, Morel PA. Regulatory Th2 response induced following adoptive transfer of dendritic cells in prediabetic NOD mice. Eur J Immunol 2002. 32(7):2021-2030. [DOD] [CrossRef]
- Papaccio G, Nicoletti F, Aurelio Pisanti F, Bendtzen K, Galdieri M. Prevention of spontaneous autoimmune diabetes in NOD mice by transferring in vitro antigen-pulsed syngeneic dendritic cells. Endocrinology 2000. 141(4):1500-1505. [DOD] [CrossRef]
- Ma L, Qian S, Liang X, Wang L, Woodward JE, Giannoukakis N, Robbins PD, Bertera S, Trucco M, Fung JJ, et al. Prevention of diabetes in NOD mice by administration of dendritic cells deficient in nuclear transcription factor-kappaB activity. Diabetes 2003. 52(8):1976-1985. [DOD] [CrossRef]
- Tacken PJ, de Vries IJM, Torensma R, Figdor CG. Dendritic-cell immunotherapy: from ex vivo loading to in vivo targeting. Nat Rev Immunol 2007. 7(10):790-802. [DOD] [CrossRef]
- Mahnke K, Guo M, Lee S, Sepulveda H, Swain SL, Nussenzweig M, Steinman RM. The dendritic cell receptor for endocytosis, DEC-205, can recycle and enhance antigen presentation via major histocompatibility complex class II-positive lysosomal compartments. J Cell Biol 2000. 151(3):673-684. [DOD] [CrossRef]
- Swiggard WJ, Mirza A, Nussenzweig MC, Steinman RM. DEC-205, a 205-kDa protein abundant on mouse dendritic cells and thymic epithelium that is detected by the monoclonal antibody NLDC-145: purification, characterization, and N-terminal amino acid sequence. Cell Immunol 1995. 165(2):302-311. [DOD] [CrossRef]
- Bonifaz L, Bonnyay D, Mahnke K, Rivera M, Nussenzweig MC, Steinman RM. Efficient targeting of protein antigen to the dendritic cell receptor DEC-205 in the steady state leads to antigen presentation on major histocompatibility complex class I products and peripheral CD8+ T cell tolerance. J Exp Med 2002. 196(12):1627-1638. [DOD] [CrossRef]
- den Haan JMM, Lehar SM, Bevan MJ. CD8+ but not CD8- dendritic cells cross-prime cytotoxic T cells in vivo. J Exp Med 2000. 192(12):1685-1696. [DOD] [CrossRef]
- Kurts C, Cannarile M, Klebba I, Brocker T. Cutting edge: dendritic cells are sufficient to cross-present self-antigens to CD8 T cells in vivo. J Immunol 2001. 166(3):1439-1442. [DOD]
- Kurts C, Kosaka H, Carbone FR, Miller JF, Heath WR. Class I-restricted cross-presentation of exogenous self-antigens leads to deletion of autoreactive CD8+ T cells. J Exp Med 1997. 186(2):239-245. [DOD] [CrossRef]
- Idoyaga J, Fiorese C, Zbytnuik L, Lubkin A, Miller J, Malissen B, Mucida D, Merad M, Steinman RM. Specialized role of migratory dendritic cells in peripheral tolerance induction. J Clin Invest 2013. In press. [DOD]
- Hawiger D, Inaba K, Dorsett Y, Guo M, Mahnke K, Rivera M, Ravetch JV, Steinman RM, Nussenzweig MC. Dendritic cells induce peripheral T cell unresponsiveness under steady state conditions in vivo. J Exp Med 2001. 194(6):769-779. [DOD] [CrossRef]
- Clynes RA, Towers TL, Presta LG, Ravetch JV. Inhibitory Fc receptors modulate in vivo cytoxicity against tumor targets. Nat Med 2000. 6(4):443-446. [DOD] [CrossRef]
- Bonifaz LC, Bonnyay DP, Charalambous A, Darguste DI, Fujii SI, Soares H, Brimnes MK, Moltedo B, Moran TM, Steinman RM. In vivo targeting of antigens to maturing dendritic cells via the DEC-205 receptor improves T cell vaccination. J Exp Med 2004. 199(6):815-824. [DOD] [CrossRef]
- Kretschmer K, Apostolou I, Hawiger D, Khazaie K, Nussenzweig MC, von Boehmer H. Inducing and expanding regulatory T cell populations by foreign antigen. Nat Immunol 2005. 6(12):1219-1227. [DOD] [CrossRef]
- Soares H, Waechter H, Glaichenhaus N, Mougneau E, Yagita H, Mizenina O, Dudziak D, Nussenzweig MC, Steinman RM. A subset of dendritic cells induces CD4+ T cells to produce IFN-g by an IL-12-independent but CD70-dependent mechanism in vivo. J Exp Med 2007. 204(5):1095-1106. [DOD] [CrossRef]
- Stylianou E, Pepponi I, van Dolleweerd CJ, Paul MJ, Ma JK, Reljic R. Exploring the vaccine potential of Dec-205 targeting in Mycobacterium tuberculosis infection in mice. Vaccine 2011. 29(12):2279-2286. [DOD] [CrossRef]
- Wang B, Zaidi N, He LZ, Zhang L, Kuroiwa J, Keler T, Steinman R. Targeting of the non-mutated tumor antigen HER2/neu to mature dendritic cells induces an integrated immune response that protects against breast cancer in mice. Breast Cancer Res 2012. 14(2):R39. [DOD] [CrossRef]
- Boscardin SB, Hafalla JC, Masilamani RF, Kamphorst AO, Zebroski HA, Rai U, Morrot A, Zavala F, Steinman RM, Nussenzweig RS, et al. Antigen targeting to dendritic cells elicits long-lived T cell help for antibody responses. J Exp Med 2006. 203(3):599-606. [DOD] [CrossRef]
- Trumpfheller C, Finke JS, Lopez CB, Moran TM, Moltedo B, Soares H, Huang Y, Schlesinger SJ, Park CG, Nussenzweig MC, et al. Intensified and protective CD4+ T cell immunity in mice with anti-dendritic cell HIV gag fusion antibody vaccine. J Exp Med 2006. 203(3):607-617. [DOD] [CrossRef]
- Maamary J, Array F, Gao Q, Garcia-Sastre A, Steinman RM, Palese P, Nchinda G. Newcastle disease virus expressing a dendritic cell-targeted HIV gag protein induces a potent gag-specific immune response in mice. J Virol 2011. 85(5):2235-2246. [DOD] [CrossRef]
- Idoyaga J, Lubkin A, Fiorese C, Lahoud MH, Caminschi I, Huang Y, Rodriguez A, Clausen BE, Park CG, Trumpfheller C, et al. Comparable T helper 1 (Th1) and CD8 T-cell immunity by targeting HIV gag p24 to CD8 dendritic cells within antibodies to Langerin, DEC205, and Clec9A. Proc Natl Acad Sci USA 2011. 108(6):2384-2389. [DOD] [CrossRef]
- Gurer C, Strowig T, Brilot F, Pack M, Trumpfheller C, Arrey F, Park CG, Steinman RM, Muenz C. Targeting the nuclear antigen 1 of Epstein-Barr virus to the human endocytic receptor DEC-205 stimulates protective T-cell responses. Blood 2008. 112(4):1231-1239. [DOD] [CrossRef]
- Iwai Y, Hemmi H, Mizenina O, Kuroda S, Suda K, Steinman RM. An IFN-g-IL-18 signaling loop accelerates memory CD8+ T cell proliferation. PLoS One 2008. 3(6):e2404. [DOD] [CrossRef]
- Do Y, Koh H, Park CG, Dudziak D, Seo P, Mehandru S, Choi JH, Cheong C, Park S, Perlin DS, et al. Targeting of LcrV virulence protein from Yersinia pestis to dendritic cells protects mice against pneumonic plague. Eur J Immunol 2010. 40(10):2791-2796. [DOD] [CrossRef]
- Tewari K, Flynn BJ, Boscardin SB, Kastenmueller K, Salazar AM, Anderson CA, Soundarapandian V, Ahumada A, Keler T, Hoffman SL, et al. Poly(I:C) is an effective adjuvant for antibody and multi-functional CD4+ T cell responses to Plasmodium falciparum circumsporozoite protein (CSP) and alphaDEC-CSP in non human primates. Vaccine 2010. 28(45):7256-7266. [DOD] [CrossRef]
- Mahnke K, Qian Y, Fondel S, Brueck J, Becker C, Enk AH. Targeting of antigens to activated dendritic cells in vivo cures metastatic melanoma in mice. Cancer Res 2005. 65(15):7007-7012. [DOD] [CrossRef]
- Wang B, Kuroiwa JM, He LZ, Charalambous A, Keler T, Steinman RM. The human cancer antigen mesothelin is more efficiently presented to the mouse immune system when targeted to the DEC-205/CD205 receptor on dendritic cells. Ann NY Acad Sci 2009. 1174(1):6-17. [DOD] [CrossRef]
- Birkholz K, Schwenkert M, Kellner C, Gross S, Fey G, Schuler-Thurner B, Schuler G, Schaft N, Doerrie J. Targeting of DEC-205 on human dendritic cells results in efficient MHC class II-restricted antigen presentation. Blood 2010. 116(13):2277-2285. [DOD] [CrossRef]
- Guo M, Gong S, Maric S, Misulovin Z, Pack M, Mahnke K, Nussenzweig MC, Steinman RM. A monoclonal antibody to the DEC-205 endocytosis receptor on human dendritic cells. Hum Immunol 2000. 61(8):729-738. [DOD] [CrossRef]
- Colonna M, Trinchieri G, Liu YJ. Plasmacytoid dendritic cells in immunity. Nat Immunol 2004. 5(12):1219-1226. [DOD] [CrossRef]
- Piccioli D, Tavarini S, Borgogni E, Steri V, Nuti S, Sammicheli C, Bardelli M, Montagna D, Locatelli F, Wack A. Functional specialization of human circulating CD16 and CD1c myeloid dendritic-cell subsets. Blood 2007. 109(12):5371-5379. [DOD] [CrossRef]
- Pack M, Trumpfheller C, Thomas D, Park CG, Granelli-Piperno A, Münz C, Steinman RM. DEC-205/CD205+ dendritic cells are abundant in the white pulp of the human spleen, including the border region between the red and white pulp. Immunology 2008. 123(3):438-446. [DOD] [CrossRef]
- Cheong C, Choi JH, Vitale L, He LZ, Trumpfheller C, Bozzacco L, Do Y, Nchinda G, Park SH, Dandamudi DB, et al. Improved cellular and humoral immune responses in vivo following targeting of HIV Gag to dendritic cells within human anti-human DEC205 monoclonal antibody. Blood 2010. 116(19):3828-3838. [DOD] [CrossRef]
- Flynn BJ, Kastenmueller K, Wille-Reece U, Tomaras GD, Alam M, Lindsay RW, Salazar AM, Perdiguero B, Gomez CE, Wagner R, et al. Immunization with HIV Gag targeted to dendritic cells followed by recombinant New York vaccinia virus induces robust T-cell immunity in nonhuman primates. Proc Natl Acad Sci USA 2011. 108(17):7131-7136. [DOD] [CrossRef]
- Hawiger D, Masilamani RF, Bettelli E, Kuchroo VK, Nussenzweig MC. Immunological unresponsiveness characterized by increased expression of CD5 on peripheral T cells induced by dendritic cells In Vivo. Immunity 2004. 20(6):695-705. [DOD] [CrossRef]
- Kretschmer K, Heng TS, von Boehmer H. De novo production of antigen-specific suppressor cells in vivo. Nat Protoc 2006. 1(2):653-661. [DOD] [CrossRef]
- Josefowicz SZ, Wilson CB, Rudensky AY. Cutting edge: TCR stimulation is sufficient for induction of Foxp3 expression in the absence of DNA methyltransferase 1. J Immunol 2009. 182(11):6648-6652. [DOD] [CrossRef]
- Schallenberg S, Tsai PY, Riewaldt J, Kretschmer K. Identification of an immediate Foxp3- precursor to Foxp3+ regulatory T cells in peripheral lymphoid organs of nonmanipulated mice. J Exp Med 2010. 207(7):1393-1407. [DOD] [CrossRef]
- Daniel C, Wennhold K, Kim HJ, von Boehmer H. Enhancement of antigen-specific Treg vaccination in vivo. Proc Natl Acad Sci USA 2010. 107(37):16246-16251. [DOD] [CrossRef]
- Petzold C, Riewaldt J, Koenig T, Schallenberg S, Kretschmer K. Dendritic cell-targeted pancreatic beta-cell antigen leads to conversion of self-reactive CD4+ T cells into regulatory T cells and promotes immunotolerance in NOD mice. Rev Diabet Stud 2010. 7(1):47-61. [DOD] [CrossRef]
- Daniel C, Weigmann B, Bronson R, von Boehmer H. Prevention of type 1 diabetes in mice by tolerogenic vaccination with a strong agonist insulin mimetope. J Exp Med 2011. 208(7):1501-1510. [DOD] [CrossRef]
- Rubtsov YP, Niec RE, Josefowicz S, Li L, Darce J, Mathis D, Benoist C, Rudensky AY. Stability of the regulatory T cell lineage in vivo. Science 2010. 329(5999):1667-1671. [DOD] [CrossRef]
- Zhou X, Bailey-Bucktrout SL, Jeker LT, Penaranda C, Martinez-Llordella M, Ashby M, Nakayama M, Rosenthal W, Bluestone JA. Instability of the transcription factor Foxp3 leads to the generation of pathogenic memory T cells in vivo. Nat Immunol 2009. 10(9):1000-1007. [DOD] [CrossRef]
- Sharma MD, Hou DY, Baban B, Koni PA, He Y, Chandler PR, Blazar BR, Mellor AL, Munn DH. Reprogrammed Foxp3+ regulatory T cells provide essential help to support cross-presentation and CD8+ T cell priming in naive mice. Immunity 2010. 33(6):942-954. [DOD] [CrossRef]
- Oldenhove G, Bouladoux N, Wohlfert EA, Hall JA, Chou D, Dos santos L, O'Brien S, Blank R, Lamb E, Natarajan S, et al. Decrease of Foxp3+ Treg Cell Number and Acquisition of Effector Cell Phenotype during Lethal Infection. Immunity 2009. 31(5):772-786. [DOD] [CrossRef]
- Tsuji M, Komatsu N, Kawamoto S, Suzuki K, Kanagawa O, Honjo T, Hori S, Fagarasan S. Preferential generation of follicular B helper T cells from Foxp3+ T cells in gut Peyer's patches. Science 2009. 323(5920):1488-1492. [DOD] [CrossRef]
- Bailey-Bucktrout SL, Bluestone JA. Regulatory T cells: stability revisited. Trends Immunol 2011. 32(7):301-306. [DOD] [CrossRef]
- Floess S, Freyer J, Siewert C, Baron U, Olek S, Polansky J, Schlawe K, Chang HD, Bopp T, Schmitt E, et al. Epigenetic control of the foxp3 locus in regulatory T cells. Plos Biol 2007. 5(2):e38. [DOD] [CrossRef]
- Polansky JK, Kretschmer K, Freyer J, Floess S, Garbe A, Baron U, Olek S, Hamann A, von Boehmer H, Huehn J. DNA methylation controls Foxp3 gene expression. Eur J Immunol 2008. 38(6):1654-1663. [DOD] [CrossRef]
- Huter EN, Punkosdy GA, Glass DD, Cheng LI, Ward JM, Shevach EM. TGF-beta-induced Foxp3+ regulatory T cells rescue scurfy mice. Eur J Immunol 2008. 38(7):1814-1821. [DOD] [CrossRef]
- Feuerer M, Hill JA, Kretschmer K, von Boehmer H, Mathis D, Benoist C. Genomic definition of multiple ex vivo regulatory T cell subphenotypes. Proc Natl Acad Sci USA 2010. 107(13):5919-5924. [DOD] [CrossRef]
- Bruder D, Westendorf AM, Hansen W, Prettin S, Gruber AD, Qian Y, von Boehmer H, Mahnke K, Buer J. On the edge of autoimmunity: T-cell stimulation by steady-state dendritic cells prevents autoimmune diabetes. Diabetes 2005. 54(12):3395-3401. [DOD] [CrossRef]
- Lo D, Freedman J, Hesse S, Palmiter RD, Brinster RL, Sherman LA. Peripheral tolerance to an islet cell-specific hemagglutinin transgene affects both CD4+ and CD8+ T cells. Eur J Immunol 1992. 22(4):1013-1022. [DOD] [CrossRef]
- Apostolou I, von Boehmer H. The TCR-HA, INS-HA transgenic model of autoimmune diabetes: limitations and expectations. J Autoimmun 2004. 22(2):111-114. [DOD] [CrossRef]
- Jasinski JM, Eisenbarth GS. Insulin as a Primary Autoantigen for Type 1A Diabetes. Clin Dev Immunol 2005. 12(3):181-186. [DOD] [CrossRef]
- Wong FS. Insulin - a primary autoantigen in type 1 diabetes? Trends Mol Med 2005. 11(10):445-448. [DOD] [CrossRef]
- Zhang L, Nakayama M, Eisenbarth GS. Insulin as an autoantigen in NOD/human diabetes. Curr Opin Immunol 2008. 20(1):111-118. [DOD] [CrossRef]
- Mukhopadhaya A, Hanafusa T, Jarchum I, Chen YG, Iwai Y, Serreze DV, Steinman RM, Tarbell KV, DiLorenzo TP. Selective delivery of beta cell antigen to dendritic cells in vivo leads to deletion and tolerance of autoreactive CD8+ T cells in NOD mice. Proc Natl Acad Sci USA 2008. 105(17):6374-6379. [DOD] [CrossRef]
- Mukherjee G, Geliebter A, Sharpe A, Freeman G, DiLorenzo T. DEC-205-mediated antigen targeting to steady-state dendritic cells induces deletion of cognate CD8+ T cells independently of the PD-1 pathway. 99th Annual Meeting of The American Association of Immunologists, Boston, MA, USA, May 4-8, 2012. [DOD]
- Weigmann B, Franke RK, Daniel C. Immunotherapy in autoimmune type 1 diabetes. Rev Diabet Stud 2012. 9(2-3):68-81. [DOD] [CrossRef]
- Wekerle H. Experimental autoimmune encephalomyelitis as a model of immune-mediated CNS disease. Curr Opin Neurobiol 1993. 3(5):779-784. [DOD] [CrossRef]
- Stern JN, Keskin DB, Kato Z, Waldner H, Schallenberg S, Anderson A, von Boehmer H, Kretschmer K, Strominger JL. Promoting tolerance to proteolipid protein-induced experimental autoimmune encephalomyelitis through targeting dendritic cells. Proc Natl Acad Sci USA 2010. 107(40):17280-17285. [DOD] [CrossRef]
- Dudziak D, Kamphorst AO, Heidkamp GF, Buchholz VR, Trumpfheller C, Yamazaki S, Cheong C, Liu K, Lee HW, Park CG, et al. Differential Antigen Processing by Dendritic Cell Subsets in Vivo. Science 2007. 315(5808):107-111. [DOD] [CrossRef]
- Loschko J, Heink S, Hackl D, Dudziak D, Reindl W, Korn T, Krug AB. Antigen targeting to plasmacytoid dendritic cells via siglec-H inhibits Th cell-dependent autoimmunity. J Immunol 2011. 187(12):6346-6356. [DOD] [CrossRef]
- Loschko J, Schlitzer A, Dudziak D, Drexler I, Sandholzer N, Bourquin C, Reindl W, Krug AB. Antigen delivery to plasmacytoid dendritic cells via BST2 induces protective T cell-mediated immunity. J Immunol 2011. 186(12):6718-6725. [DOD] [CrossRef]
- Caminschi I, Proietto AI, Ahmet F, Kitsoulis S, Shin Teh J, Lo JC, Rizzitelli A, Wu L, Vremec D, van Dommelen SL, et al. The dendritic cell subtype-restricted C-type lectin Clec9A is a target for vaccine enhancement. Blood 2008. 112(8):3264-3273. [DOD] [CrossRef]
- Castro FV, Tutt AL, White AL, Teeling JL, James S, French RR, Glennie MJ. CD11c provides an effective immunotarget for the generation of both CD4 and CD8 T cell responses. Eur J Immunol 2008. 38(8):2263-2273. [DOD] [CrossRef]
- Carter RW, Thompson C, Reid DM, Wong SY, Tough DF. Preferential induction of CD4+ T cell responses through in vivo targeting of antigen to dendritic cell-associated C-type lectin-1. J Immunol 2006. 177(4):2276-2284. [DOD]
- Tagliani E, Guermonprez P, Sepulveda J, Lopez-Bravo M, Ardavin C, Amigorena S, Benvenuti F, Burrone OR. Selection of an antibody library Identifies a pathway to induce immunity by targeting CD36 on steady-state CD8+ dendritic cells. J Immunol 2008. 180(5):3201-3209. [DOD]
- Henri S, Poulin LF, Tamoutounour S, Ardouin L, Guilliams M, de Bovis B, Devilard E, Viret C, Azukizawa H, Kissenpfennig A, et al. CD207+ CD103+ dermal dendritic cells cross-present keratinocyte-derived antigens irrespective of the presence of Langerhans cells. J Exp Med 2009. 207(1):189-206. [DOD] [CrossRef]
- Culina S, Boitard C, Mallone R. Antigen-based immune therapeutics for type 1 diabetes: magic bullets or ordinary blanks? Clin Dev Immunol 2011. 2011:286248. [DOD]
- Ejaz A, Ammann CG, Werner R, Huber G, Oberhauser V, Horl S, Schimmer S, Dittmer U, von Laer D, Stoiber H, et al. Targeting viral antigens to CD11c on dendritic cells induces retrovirus-specific T cell responses. Plos One 2012. 7(9):e45102. [DOD] [CrossRef]
- Singh SK, Stephani J, Schaefer M, Kalay H, Garcia-Vallejo JJ, den Haan J, Saeland E, Sparwasser T, van Kooyk Y. Targeting glycan modified OVA to murine DC-SIGN transgenic dendritic cells enhances MHC class I and II presentation. Mol Immunol 2009. 47(2-3):164-174. [DOD] [CrossRef]
- Tacken PJ, de Vries IJM, Gijzen K, Joosten B, Wu D, Rother RP, Faas SJ, Punt CJA, Torensma R, Adema GJ, et al. Effective induction of naive and recall T-cell responses by targeting antigen to human dendritic cells via a humanized anti-DC-SIGN antibody. Blood 2005. 106(4):1278-1285. [DOD] [CrossRef]
This article has been cited by other articles:
|
Multiple sclerosis: Therapeutic applications of advancing drug delivery systems
Dolati S, Babaloo Z, Jadidi-Niaragh F, Ayromlou H, Sadreddini S, Yousefi M
Biomed Pharmacother 2017. 86:343-353
|
|
|
Targeting CLEC9A delivers antigen to human CD141+ DC for CD4+ and CD8+T cell recognition
Tullett KM, Leal Rojas IM, Minoda Y, Tan PS, Zhang JG, Smith C, Khanna R, Shortman K, Caminschi I, Lahoud MH, Radford KJ
JCI Insight 2016. 1(7):e87102
|
|
|
DEC205+ Dendritic Cell-Targeted Tolerogenic Vaccination Promotes Immune Tolerance in Experimental Autoimmune Arthritis
Spiering R, Margry B, Keijzer C, Petzold C, Hoek A, Wagenaar-Hilbers J, van der Zee R, van Eden W, Kretschmer K, Broere F
J Immunol 2015. 194(10):4804-4813
|
|
|
Advancing drug delivery systems for the treatment of multiple sclerosis
Tabansky I, Messina MD, Bangeranye C, Goldstein J, Blitz-Shabbir KM, Machado S, Jeganathan V, Wright P, Najjar S, Cao Y, Sands W, Keskin DB, Stern JN
Immunol Res 2015. 63(1-3):58-69
|
|
|
Transdermal delivery of bovine milk vesicles in patients with multiple sclerosis: A novel strategy to induce MOG-specific tolerance
Mokarizadeh A, Hassanzadeh K, Abdi M, Soraya H, Faryabi MR, Mohammadi E, Ahmadi A
Med Hypotheses 2015. 85(2):141-144
|
|
|
Regulatory dendritic cells for immunotherapy in immunologic diseases
Gordon JR, Ma Y, Churchman L, Gordon SA, Dawicki W
Front Immunol 2014. 5:7
|
|
|