Chapter II. Prevention
Rev Diabet Stud,
2012,
9(4):260-271 |
DOI 10.1900/RDS.2012.9.260 |
Virus Infections as Potential Targets of Preventive Treatments for Type 1 Diabetes
Noora Nurminen1, Sami Oikarinen1, Heikki Hyöty1,2
1Department of Virology, School of Medicine, University of Tampere, Tampere, Finland
2Fimlab Laboratories, Pirkanmaa Hospital District, Tampere, Finland
Address correspondence to: Heikki Hyoty, Department of Virology, School of Medicine, University of Tampere, Biokatu 10, 33520 Tampere, Finland, e-mail: heikki.hyoty@uta.fi
Manuscript submitted November 28, 2012; resubmitted January 21, 2013; accepted January 28, 2013.
Keywords: type 1 diabetes, enterovirus, coxsackie B virus, pathogenesis, environment, virus, infection
Abstract
Environmental factors play an important role in the pathogenesis of type 1 diabetes, and are attractive targets for preventive interventions. Several studies have shown that viruses can cause diabetes in animals, indicating their potential as candidates for environmental triggering agents. However, human studies have been hampered by the complex nature of the disease pathogenesis, leaving the question of viral etiology unanswered. Significant progress has recently been made in this field by searching for viruses within pancreatic tissue samples, and by carrying out prospective studies. Consequently, there is increasing evidence for a group of enteroviruses acting as possible environmental key triggers. In past studies, these viruses have been linked to type 1 diabetes. Recent studies have shown that they exert tropism to pancreatic islets, and that they are associated with the start of the beta-cell damaging process. Also, polymorphisms of the gene coding for the innate immune system sensor for enteroviruses (IFIH1) were found to modulate the risk of diabetes. Based on these findings, interest in the possible development of vaccines against these viruses has increased. However, even if enterovirus vaccines (polio vaccines) are effective and safe, we currently lack necessary information for the development of a vaccine against diabetogenic enteroviruses, e.g. regarding the identification of their specific serotypes and the causal relationship between these viruses and diabetes initiation. Ongoing research projects are currently addressing these questions, and will hopefully increase the consensus in this field. Also, new sequencing technologies will provide additional information about the whole virome, which could enable the discovery of new candidate viruses.
Abbreviations: CBV – coxsackie B virus; EV – enterovirus; IFIH1 – interferon induced with helicase C domain 1; nPOD – Network for Pancreatic Organ Donors with Diabetes; PEVNET – Persistent Virus Infection in Type 1 Diabetes (study); RNA – ribonucleic acid; PCR – polymerase chain reaction; T1D – type 1 diabetes; TEDDY - The Environmental Determinants of Diabetes in the Young (study)
1. Background
Environmental factors are known to have a significant effect on the risk of type 1 diabetes (T1D), suggesting their important role in T1D pathogenesis [1]. In spite of this knowledge, it has been difficult to identify specific risk or protective factors that are responsible for this environmental effect. Viruses have long been among the major candidates and have been associated with T1D in several studies.
The interest in viruses originally resulted from the observation that they caused a T1D-like disease in animals. The first observations were done in virus epidemics in cows, which led to diabetes in the infected animals [2]. During the last few decades, several different animal models have been developed to study the mechanisms of virus-induced diabetes. These studies have shown that several viruses have an ability to cause diabetes in animals by various mechanisms [3-5].
Human studies have been complicated by the presence of several non-viral factors that modulate the risk of T1D and that are difficult to control in case-control studies. An additional problem is that beta-cell damage usually progresses slowly. This fact challenges the identification of viruses that have started the process leading to T1D long before its clinical diagnosis. Prospective studies can largely overcome this problem, but the detection of viruses, which exist only transiently and which represent a small subgroup of a larger family of viruses commonly circulating in the human population, is methodologically demanding. In spite of these challenges, a considerable amount of evidence has accumulated supporting the diabetogenic effect of certain viruses in human T1D.
The unveiling of the viral etiology of T1D is important. If causal viruses exist, their identification could establish a direct and feasible avenue for the prevention of T1D through the production of vaccines. Virus vaccines have been among the greatest success stories in medicine, providing a safe and effective way for the prevention of many viral diseases. The strongest evidence for viral etiology of human T1D has been obtained from studies addressing the role of enteroviruses (EVs) [6, 7]. Therefore, this review focuses on the group of EVs and the current scenarios of developing vaccines against it.
2. Lessons from other enterovirus diseases and the pathogenesis of type 1 diabetes
Human EVs are the most common human viruses. They are small RNA viruses, which include more than 100 different genotypes; polioviruses being the best-characterized examples. Also, rhinoviruses are currently classified as EVs due to their genetic similarities. Primary replication happens in the lymphatic tissues of the oropharynx and intestine [8]. The infection may be restricted to mucosal surfaces, but in some cases the virus invades the body and spreads to circulation causing viremia. While most EV infections are mild or asymptomatic, they can also lead to severe and even life-threatening manifestations. Typical features of severe disease include systemic spread of the virus into the blood and certain inner organs, leading to disturbed organ function that causes the symptoms of the disease. The target organ that becomes infected depends on the tropism of the virus imposed on certain cell types, a phenomenon which is largely linked to the serotype of the virus and the binding of the virus to specific cellular receptors [9, 10].
An observed low attack rate indicates that the cases with severe disease represent only the tip of the iceberg, and most infections remain unrecognized. Examples of severe EV diseases include myocarditis, myositis, encephalitis, meningitis, and paralysis (Table 1). Polio has been used as a model disease, and its pathogenesis has been studied widely. Three EV types have been named as polioviruses (poliovirus types 1, 2, and 3) due to their ability to cause polio paralysis. This serotype restriction is reflected by the tropism of these particular virus types exerted to the anatomical site of the pathogenesis (central nervous system and spinal cord). However, it is not known why these viruses so selectively destroy one particular cell type (motor neurons) [11]. This kind of highly cell-specific damage is explained by the expression of poliovirus receptors (CD 155) on target cells and the involvement of other factors regulating the replication of the virus in different cell types. In any case, it seems that motor neurons are directly infected by the virus, and infections cause inflammation and infiltration of immune cells into the infected tissue which may also contribute to cell damage. The degree of paralysis varies from mild and transient to severe and permanent paralysis of the whole limb. One striking feature of polio is the extremely low attack rate: less than one per cent of infected individuals develop paralysis. The factors regulating the development of this severe form of the disease are poorly characterized, but the risk is increased by factors such as male gender, humoral immunodeficiency, extensive exercise, intramuscular injections, and adenoidectomy prior/during the infection.
Table
1.
Examples of enterovirus diseases |
|
 |
 |
In addition to acute infections, EVs can also cause persistent infections. Viral persistence is often associated with immune-mediated damage in target organs. Chronic cardiomyopathy represents such condition in man [12]. In mouse models, persistent EV infection causes inflammatory myopathies, cardiac injury, and central nervous system damage [13, 14]. Immunocompromised patients, especially those with humoral immunodeficiency, also suffer from chronic EV infections. General characteristics of EV disease are summarized in Table 2.
Table
2.
Key characteristics of enterovirus disease |
|
 |
 |
If EVs cause T1D, it is logical to assume that both conditions follow similar pathogenetic features, as known from other EV diseases. However, in contrast to poliovirus-induced neuronal damage, where even a small number of damaged cells can cause immediate symptoms, a minor reduction in the number of insulin-producing cells would remain unrecognized, as the remaining beta-cells would be able to compensate for the deficiency to maintain glucose tolerance. This impedes the determination of the time relationship between causative infection and beta-cell damage. In addition, latest evidence supports the hypothesis that EV infections manifest in a slow rate of replication and persistence rather than an acute infection. Thus, the detection of EVs during T1D progression requires highly sensitive techniques. As in polio, the diabetogenic property may be linked to a few specific EV serotypes that would have exerted tropism to human pancreatic islets and especially to insulin-producing beta-cells. The fact that EV proteins and genomes have been detected almost exclusively in pancreatic islets and not in exocrine tissue supports this tropism hypothesis [15-17]. This means that the identification of possible diabetogenic effect of EVs would require assays that can distinguish diabetogenic virus types from other abundant EV types. In addition, mutations in the viral genome may further modulate the diabetogenicity in the same way as the neurovirulence of polioviruses is modulated by certain nucleotide changes in the coding and non-coding regions of the viral genome.
Even though many aspects of the pathogenesis are still unexplained, the existing evidence supports the role of direct infection in the pancreatic islets. Such an infection can damage beta-cells by an acute cytolytic effect and by a slower immune-mediated damage. The latter component can be mediated by the activation of the innate immune system causing local inflammation and by adaptive immune responses, which can first be induced against virus-infected cells, but expand later to islet autoantigens. This kind of epitope spreading from viral antigens to islet autoantigens is likely if the virus persists in the islets causing long-lasting inflammation (induction of autoimmune responses happens in many chronic virus diseases). In addition, other immune-mediated mechanisms can contribute to the cell damage. Such mechanisms include immunological cross-reactivity between viral and host antigens (molecular mimicry) and possible induction of non-neutralizing anti-viral "enhancing" antibodies, which can lead to strong immune activation and worsen the pathogenesis [7, 18-20]. A hypothetical model of some key determinants of EV diabetogenicity is shown in Figure 1.
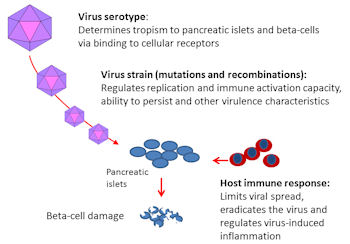 |
 |
Figure 1. Viral and host factors regulating the risk of virus-induced beta-cell damage. The serotype of enteroviruses is a key determinant of viral tropism exerted to different organs such as pancreatic islets since it is connected to the specific binding of the virus to cell receptors. Furthermore, each serotype is represented by a variety of virus strains developed as a result of single-nucleotide mutations and genomic recombinations, resulting in different virulence characteristics. The host immune response controls the virus spread and generates inflammation in infected tissues such as the pancreas. |
|
3. How potential diabetogenic effects of enteroviruses can be detected in human studies
The possible role of EVs in T1D has been evaluated in numerous case-reports, case-control studies, and prospective studies by measuring antibody responses against EVs or by detecting the virus directly in the study subjects. Different study designs have their own advantages and disadvantages. Several aspects should be considered when designing such studies. The most important aspects are briefly described below.
3.1 Role of non-invasive and invasive infection
As described above, severe EV manifestations are related to invasive infections, when the virus spreads widely in the body, such that different organs can be infected (Figure 2). Accordingly, the detection of invasive infections may be important for the identification of the diabetogenic effects of EVs. Studies detecting EVs in the blood or pancreas could give better risk estimates than studies evaluating only the general exposure to the virus. Serological studies and studies detecting viruses in respiratory or stool samples detect exposure, but not invasion (Figure 2). In fact, this seems to be the case, since serological studies and the detection of EVs from stool samples have indicated only weak or no risk effect [21-23]. In contrast, studies based on virus detection from blood or pancreas have shown a risk effect [24, 25].
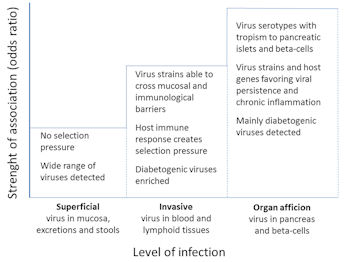 |
 |
Figure 2. Identification of diabetogenic enterovirus types. The figure shows a hypothetical model of the probability to identify diabetogenic enterovirus types from different kinds of samples according to the level of infection. The probability is highest (i.e. it has the highest odds ratio) when samples are collected directly from the pancreas since virus types infecting the target organ have passed several anatomical and immunological barriers, being enriched with causative virus types and strains. In contrast, infections diagnosed by virus detection from stools or by antibody assays from serum mostly include non-diabetogenic enteroviruses, and the diabetogenic viruses represent only a small fraction of all virus types detected in type 1 diabetic patients. |
|
3.2 The low attack rate of enteroviruses
As described above, the attack rate of EVs is generally low. In the case of polio, the virus was very common, circulating widely in the population, and leading to the paralytic complication in less than 1% of all infected individuals. This led to high antibody prevalence in the background population; in many countries the great majority of adults had antibodies against polioviruses [26]. If this is also true for diabetogenic EVs, the frequency of virus antibodies should show only a modest difference between T1D patients and controls, making it difficult to identify the diabetogenic effect of EVs (Figure 3). The fact that primary beta-cell insult is asymptomatic and occurs long before clinical T1D further increases this challenge.
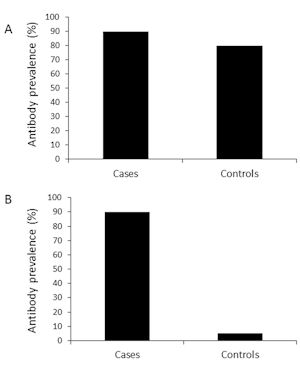 |
 |
Figure 3. Prevalence of virus antibody. The figure shows a hypothetical model of virus antibody prevalence seen in case-control studies in two-virus diseases with either low (A) or high (B) attack rate. Patients with severe enterovirus disease have only slightly higher antibody prevalence than control subjects due to the low attack rate (only <1% of poliovirus-infected individuals got paralysis) (A). A similar figure is shown for a virus disease that is characterized by a high attack rate (such as HIV-induced AIDS) (B). |
|
3.3 Importance of distinguishing EV subtypes from each other
It is important to distinguish EV subtypes if only some EV types are diabetogenic (analogous to polio and other EV diseases). Most currently available antibody assays detect antibodies against a wide range of different EV types due to the presence of common group-reactive antigen epitopes in viral proteins. Such assays are biased by "background noise" caused by antibodies induced by all non-diabetogenic EVs, which can mask antibodies induced by diabetogenic EV types. One can claim that the link between polioviruses and paralysis would have never been discovered in retrospective case-control studies if such non-serotype-specific antibody assays had been used. Also, most polymerase chain reaction (PCR) assays have been based on primers that amplify conserved regions of EVs and that do not allow the identification of virus subtypes. Therefore, the use of serotype-specific assays offers an important advantage in the evaluation of the causal relationship between EVs and T1D. Currently, there are three methods available:
1. Measurement of neutralizing antibodies in serum
2. Isolation of the virus, which also allows detailed typing of the virus
3. Sequencing of the viral genome directly from clinical samples
3.4 Possible viral persistence
Persisting EV infections are characterized by a slowly replicating virus strain which may contain specific deletions in its genome [12]. This kind of persisting virus seems to be involved in EV-induced cardiomyopathy, and it has been suspected in T1D as well. The focus of persisting EV can be in the pancreas, or as suggested in recent studies, in the intestinal mucosa [27]. Highly sensitive methods are needed to detect such infections due to the slow replication rate of the virus. Also, it is detectable only in tissue samples such as pancreas, but not in serum. The need for high methodological sensitivity is further emphasized by studies suggesting that only a very small proportion of pancreatic cells are infected by EV in T1D patients. This proportion can be as low as 0.01% (personal communication with Gun Frisk, University of Uppsala, Sweden). Infected cells are mainly islet cells. Some studies have indicated that the infected cells are insulin-positive beta-cells [28]. It seems that EV can indeed be difficult to find in infected tissues. For example, in EV71 encephalitis, the virus proteins and RNA were detected only in a small number of neurons and in some phagocytic cells [29]. The isolation of pancreatic islets prior to virus detection may increase the success rate of EV detection in T1D.
3.5 Role of host's susceptibility factors
Several factors regulate the host's susceptibility to EV infections, and can possibly modulate their diabetogenic effect. General risk factors for severe EV disease include young age, male gender, humoral immunodeficiency, lack of maternal antibodies, and short duration of breast-feeding [30, 31]. In addition, certain genes, whose polymorphisms have been linked to T1D, are related to immune responses against EVs. One such gene is IFIH1 that codes for an innate immune system receptor for EVs [32]. It has been proposed that the T1D-associated IFIH1 genotype is associated with a strong anti-viral response causing a deleterious inflammation process in the infected tissue [33, 34].
3.6 Population effect
Analogous to polio, the diabetogenic effect of EVs may vary between populations. Poliovirus infection led to paralysis most frequently in countries with a high standard of hygiene [11]. This phenomenon was related to the balance between age-related host susceptibility and age of first infections. In prosperous countries, the circulation of polioviruses was at a relatively low level due to the high standard of hygiene. In such an environment a relatively large proportion of children got their first infections at an older age when they were no longer protected by maternal antibodies. Moreover, maternal antibodies were at lower levels than in populations with a high frequency of infections. Thus, the diabetogenic effect caused by EVs may be characterized analogously to the "polio hypothesis", which describes that the effects of EV infections are strongest in countries where the viruses are relatively rare [35]. In fact, ecological comparisons between different countries have suggested that the frequency of EV infections in the background population shows an inverse correlation with the incidence of T1D [36]. Increasing incidence of T1D seems to be associated with decreasing frequency of EV infections over time [35, 37]. The diabetogenic effect caused by EVs has also been repeatedly observed in Finland, where EV infections are less common than in other European countries, but T1D incidence is at maximum.
3.7 Recruitment of study cohorts
The study design has a fundamental effect on the detection of EV infections in the study subjects. Direct virus detection methods are sensitive for the timing of sampling. For example in blood, the virus may be detectable only for a few days. Therefore, retrospective case-control studies may fail to detect diabetogenic infections that have most likely occurred years earlier, unless the virus persists, rendering the patients chronically virus-positive. Such studies have also limited value in serological surveys, particularly if the assays do not distinguish diabetogenic EV types from non-diabetogenic EV types. Prospective studies that follow originally non-diabetic individuals, covering the time when the beta-cell damaging process is initiated, offer the best option to study the role of EVs in T1D (e.g. birth-cohort studies). Frequent sample collection and a wide range of different kinds of samples make it possible to use both direct virus detection methods and antibody assays to diagnose EV infections [38]. The criteria used for the selection of control subjects are also critical for the study.
3.8 Detecting diabetogenic effects of EVs - summary
In summary, the evaluation of possible diabetogenic effects of EVs is a challenging task. Epidemiological studies need careful designing, taking into account all the aspects described in sections 3.1 to 3.7 (Figure 2). Optimal studies are technically and scientifically challenging and require significant financial support. They should be able to detect an invasive infection (blood or tissue samples collected), identify the exact type of EV, and include different populations. The combined application of direct virus detection and antibody measurement provides an optimal basis for the reliable diagnosis of EV infections. For example, the measurement of neutralizing antibodies enables the diagnosis of both acute and past infections (serological scar) as well as the type of EV causing the infection.
It is also helpful to measure T cell and innate immune system responses. This method can help to diagnose infections and provide information about the host’s response to the virus. The emerging next-generation sequencing technologies offer exciting opportunities for virus discovery. International collaboration is needed to carry out studies in different populations. Currently, the largest ongoing initiatives include The Environmental Determinants of Diabetes in the Young (TEDDY) study (prospective birth cohort study evaluating environmental causes of T1D; http://teddy.epi.usf.edu), the Network for Pancreatic Organ Donors with Diabetes (nPOD) study (collection of pancreatic tissues from T1D patients; http://www.jdrfnpod.org/), and the Persistent virus infection in type 1 diabetes (PEVNET) study (large biobank survey to identify diabetogenic EVs and mechanisms of their action; http://www.uta.fi/med/ pevnet/index.html).
4. How to prove causality?
It is difficult to prove or disprove causality between infections and chronic diseases, especially if the existing paradigm does not support infectious etiology. This has been experienced in research evaluating the role of infections in the development of various cancer types; it took decades to reach general agreement about the causal relationship between e.g. helicobacteria, papillomavirus, hepatitis B virus, or Epstein-Barr virus and cancer, even if these infections are currently considered as the major causative agents in gastric cancer, cervical cancer, hepatocellular cancer, and certain lymphomas, respectively [39-42].
Possible causal links between EVs and T1D will obviously need equally strong and definite proof to become accepted. According to published meta-analyses, the association between EV and T1D is quite strong in studies which have been based on direct virus detection from blood or tissues (odds ratios ranging from 4 to 10) [24]. However, serological studies have shown more variable results [21], possibly because they measure the general exposure and not invasive infection. The discovery of IFIH1 as a risk gene for T1D has recently offered a feasible mechanistic explanation for this association [32, 34]. Other support comes from ecological studies (polio hypothesis) [35], in vitro models (EV infection in islet cell cultures) [43], and mouse models of EV-caused diabetes [3]. However, the general consensus is that the currently existing evidence is not strong enough to prove causal relationship. There is a need for additional, convincing evidence, which could be based on the following kind of data:
1. Confirmation of the diabetogenic effect of EVs in large epidemiological studies (preferentially prospective studies carried out in different populations)
2. Demonstration of EVs in the pancreatic islets in a large series of T1D patients by different technologies
3. Identification of viral determinants of diabetogenicity (such as serotype or mutations in viral genome) and host factors regulating susceptibility to the viral effect
The final proof of causality would need intervention trials showing that the elimination of EVs can reduce the risk of T1D. The following three ways of intervention could be considered:
1. Vaccines
2. Antiviral drugs
3. Hyper-immune sera
Vaccines offer one of the most attractive opportunities for such interventions since they can induce effective and long-term protection against EVs, as shown by the widely used poliovirus vaccines. However, the development of a new vaccine is a highly expensive enterprise. Antiviral drugs could offer an alternative opportunity, but such interventions should be targeted to autoantibody positive children with already ongoing beta-cell damaging process assuming that the process is driven by a persisting EV infection in the pancreas. Treatment with antiviral drugs may also generate resistant virus strains. A few drugs that are effective against EVs are currently available (e.g. interferon-alpha, ribavirin). Interferon-alpha has been tested in newly diagnosed T1D patients with varying effects on beta-cell function [44, 45]. Finally, hyper-immune sera against EVs could be considered. Such a treatment was successfully used to prevent poliomyelitis before polio vaccines were developed.
5. Current scenarios in the development of EV vaccines to treat type 1 diabetes
The technologies to produce EV vaccines are available. In addition to the widely used poliovirus vaccines, new vaccines against enterovirus 71 are tested in clinical trials, and several other EV vaccines have been used effectively in animal models [46]. Based on these possibilities, one may ask why such vaccines have not been tested in the prevention of T1D. However, there are several open questions which need to be answered before the possible preventive effect of new EV vaccines can be tested in clinical trials. These questions are summarized below.
5.1 Type of diabetogenic EVs
One of the key questions is related to the identification of the exact types of diabetogenic EVs. A possible vaccine should include these virus types to be effective. The identification of the diabetogenic EVs would also enable the study of mechanisms of virus-induced diabetes by analyzing how these virus types differ from other EVs. Unfortunately, only few studies have focused on the identification of these virus types.
Early studies in the UK suggested that the group of six coxsackie B viruses (CBVs), and especially the CBV4 serotype, may be important; neutralizing antibodies against this virus were increased in T1D patients [47, 48]. CBVs have also been isolated from the pancreas of T1D patients on two occasions (both were CBV4) [28, 49]. Autopsy studies among children who died of CBV infection have indicated islet cell damage and insulitis in their pancreas, while no damage was seen in infections caused by other EV serotypes [50]. CBVs can cause diabetes and pancreatitis in mouse models and in monkeys [3, 51]. Also, CBV1 was the only EV type associated with T1D risk in a recent prospective study which was based on systematic screening of neutralizing antibodies against several different EV serotypes in Finland [52]. This study showed that CBV3 had a protective effect indicative of immunological cross-protection against CBV1 [53]. In another recent study, performed in different European populations, CBV1 was again associated with increased risk of T1D [54]. In addition, some EVs other than CBVs (mainly certain echoviruses) have been linked to T1D in some studies [55]. Altogether, one can conclude that CBVs are currently the chief candidates for diabetogenic EV types in man. Therefore, a multivalent vaccine containing CBV serotypes and possibly some echoviruses would be a good option for a vaccine to be tested in clinical trials.
5.2 Safety of the vaccine
The target group for the vaccine includes healthy young children. Therefore, the safety of the vaccine is of high priority. The long experience from poliovirus vaccines suggests that these vaccines are safe. Two kinds of poliovirus vaccines are in the market. The inactivated vaccine (Salk’s vaccine) includes all three poliovirus types which have been inactivated by formalin to destroy their infectivity. This vaccine is one of the safest vaccines ever developed, having no significant side-effects. The live attenuated vaccine (Sabin's vaccine) includes infective polioviruses, but its neurovirulence has been attenuated by serial passages in cell cultures. This vaccine is also safe, but in very rare occasions the vaccine virus reverts back towards the neurovirulent phenotype, causing paralysis in vaccine recipients and their contacts.
In addition to general safety aspects, possible T1D-specific safety issues should be carefully considered. Theoretically, the vaccine could cause beta-cell damage if it shares common antigenic structures with beta-cell proteins (molecular mimicry). Previous studies have suggested that such epitopes may exist both in beta-cell and EV proteins, but their role in the pathogenesis of T1D remains elusive [20]. Inactivated CBV vaccine does not exaggerate the development of diabetes in NOD mouse, and there are no indications that polio vaccines could cause diabetes [56]. It is thus unlikely that the vaccine would cause T1D, but this safety aspect should be carefully considered in all states of a vaccine development program.
5.3 Strength of evidence
The third important aspect is the confidence level that needs to be reached before the clinical development of a vaccine can be started. The evidence for causality should be quite robust because of the high costs of a clinical development program. We recall the analogy to the human papilloma viruses and their role in cervical cancer; it took a long time before the required confidence level was reached and the development of vaccines could be started.
5.4 T1D susceptibility attributable to EV infections
It is important to determine what proportion of T1D susceptibility can be causally attributed to EVs. Based on current knowledge, this fraction may account for 50% or more, but it is difficult to make reliable estimations. The main reason is that most studies have been based on methods that cannot distinguish EV serotypes from each other. If only some of them are diabetogenic the true proportion of virus-induced T1D cases is hard to determine.
5.5 Current stage of development of an EV vaccine
Currently, initial efforts to explore the opportunities for the development of an EV vaccine against T1D are underway. These efforts are based on an international consortium that includes academic partners and vaccine companies. The project is aimed at the identification of diabetogenic EV serotypes in different populations and testing prototype vaccines in mouse models. It is too early to conclude whether this effort will lead to clinical trials. In any case, it will provide important information about the role of EVs in T1D and the possibilities of developing safe and effective EV vaccines for primary prevention of the disease.
The existing live poliovirus vaccines may also provide a protective effect against non-polio EVs by immunological cross-protection and viral interference. Even if these vaccines do not provide complete protection against non-polio EVs, it is possible that they can protect against severe infections and systemic spread of the virus. Memory T cells that have been induced by polioviruses (or poliovirus vaccines) can cross-react with non-polio EVs [57] and boost immune responses during later EV infections. Live poliovirus vaccine induces stronger T cell responses than inactivated poliovirus vaccines. For a long time, countries with high T1D incidence (e.g. Finland and Sweden) have used inactivated poliovirus vaccines, while others have used live poliovirus vaccines. A recent study suggests that live poliovirus vaccine protects against otitis media, a finding which would support the hypothesis of immunological cross-protection against non-polio EVs causing otitis media [58]. The possible protective effect of live poliovirus vaccines against T1D has never been tested in clinical trials.
6. Conclusions
Research on the possible viral etiology of T1D is in a very active stage, and several large-scale studies are in progress. Certain key research areas have been identified, with the potential of filling critical gaps in current knowledge (Table 3). Large epidemiological studies should be carried out to confirm the risk effect of EVs, especially that of specific EV types (e.g. CBVs), in different populations, and to provide reliable estimates for the proportion of T1D susceptibility caused by EVs (attributable fraction). Furthermore, new studies evaluating the presence of EVs in the pancreas of T1D patients would provide important additional information about the role of EV infections in T1D and their structural characteristics.
Table
3.
Research items and actions to augment the possible development of enterovirus vaccines against type 1 diabetes |
|
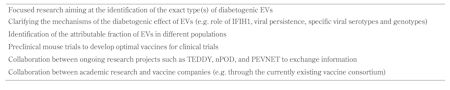 |
 |
The role of the IFIH1 gene, the innate immune system and viral persistence are examples of other important areas of research. Preclinical vaccine studies would be needed to develop optimal vaccine constructs for clinical trials. Each approach of intervention for the elimination of EV infections in the pancreas has its weaknesses and strengths. Multiple studies carried out in different populations are needed to achieve a confidence level necessary to determine the role of EV infections in the pathogenesis of T1D. Based on this possible future evidence, clinical vaccine development could be started, or alternatively, it may be concluded that EVs do not play a significant role in the pathogenesis of T1D.
It should also be considered that other viruses beside EVs may have a possible role in T1D, even if EVs are currently the main focus. Next-generation sequencing technologies offer an attractive opportunity for studying other viruses in addition to EVs. They are currently used to characterize the whole virome of T1D patients, e.g. in the prospective TEDDY study. It would be important to standardize these new technologies for their sensitivity and reproducibility to make them comparable between studies and applicable in a safely repeatable manner.
Disclosure: Heikki Hyöty is a minor (<5%) shareholder and member of the board of Vactech Ltd., which develops vaccines against picornaviruses.
Acknowledgments:
We wish to thank Hanna Honkanen, MSc, Maarit Oikarinen, PhD, Anita Kondrashova, MD, PhD, Amir-BabakSioofy-Khojine, PhD, SiskoTauriainen, PhD, Maria Lönnrot, MD, PhD, Olli Laitinen, PhD, Mikael Knip, MD, PhD, and all other collaborators contributing to the findings and discussions summarized in this review. This work was supported by the Competitive Research Funding of the Tampere University Hospital, the Juvenile Diabetes Research Foundation (JDRF), the Academy of Finland, and the European Commission (PEVNET FP-7 Programme, Contract No. 261441).
References
- Knip M, Veijola R, Virtanen SM, Hyoty H, Vaarala O, Akerblom HK. Environmental triggers and determinants of type 1 diabetes. Diabetes 2005. 54(Suppl 2):S125-S136. [DOD] [CrossRef]
- Barboni E, Manocchio J. Alterazioni pancreatiche in bovini con diabete mellito post-aftoso. Arch Vet Ital 1962. 13:477-489. [DOD]
- Jaidane H, Sane F, Gharbi J, Aouni M, Romond MB, Hober D. Coxsackievirus B4 and type 1 diabetes pathogenesis: contribution of animal models. Diabetes Metab Res Rev 2009. 25:591-603. [DOD] [CrossRef]
- Tracy S, Drescher KM. Coxsackievirus infections and NOD mice: relevant models of protection from, and induction of, type 1 diabetes. Ann NY Acad Sci 2007. 1103:143-151. [DOD] [CrossRef]
- Yoon JW, Jun HS. Viruses cause type 1 diabetes in animals. Ann NY Acad Sci 2006. 1079:138-146. [DOD] [CrossRef]
- Coppieters KT, Boettler T, von Herrath M. Virus infections in type 1 diabetes. Cold Spring Harb Perspect Med 2012. 2:a007682. [DOD] [CrossRef]
- Hober D, Sauter P. Pathogenesis of type 1 diabetes mellitus: interplay between enterovirus and host. Nat Rev Endocrinol 2010. 6:279-289. [DOD] [CrossRef]
- Racaniello VR. One hundred years of poliovirus pathogenesis. Virology 2006. 344:9-16. [DOD] [CrossRef]
- Palacios G, Oberste MS. Enteroviruses as agents of emerging infectious diseases. J Neurovirol 2005. 11:424-433. [DOD] [CrossRef]
- Tapparel C, Siegrist F, Petty TJ, Kaiser L. Picornavirus and enterovirus diversity with associated human diseases. Infect Genet Evol 2012. In press. [DOD]
- Mueller S, Wimmer E, Cello J. Poliovirus and poliomyelitis: a tale of guts, brains, and an accidental event. Virus Res 2005. 111:175-193. [DOD] [CrossRef]
- Chapman NM, Kim KS. Persistent coxsackievirus infection: enterovirus persistence in chronic myocarditis and dilated cardiomyopathy. Curr Top Microbiol Immunol 2008. 323:275-292. [DOD] [CrossRef]
- Tam PE, Schmidt AM, Ytterberg SR, Messner RP. Duration of virus persistence and its relationship to inflammation in the chronic phase of coxsackievirus B1-induced murine polymyositis. J Lab Clin Med 1994. 123:346-356. [DOD]
- Feuer R, Ruller CM, An N, Tabor-Godwin JM, Rhoades RE, Maciejewski S, Pagarigan RR, Cornell CT, Crocker SJ, Kiosses WB, et al. Viral persistence and chronic immunopathology in the adult central nervous system following Coxsackievirus infection during the neonatal period. J Virol 2009. 83:9356-9369. [DOD] [CrossRef]
- Richardson SJ, Willcox A, Bone AJ, Foulis AK, Morgan NG. The prevalence of enteroviral capsid protein vp1 immunostaining in pancreatic islets in human type 1 diabetes. Diabetologia 2009. 52:1143-1151. [DOD] [CrossRef]
- Oikarinen M, Tauriainen S, Honkanen T, Vuori K, Karhunen P, Vasama-Nolvi C, Oikarinen S, Verbeke C, Blair GE, Rantala I, et al. Analysis of pancreas tissue in a child positive for islet cell antibodies. Diabetologia 2008. 51:1796-1802. [DOD] [CrossRef]
- Ylipaasto P, Klingel K, Lindberg AM, Otonkoski T, Kandolf R, Hovi T, Roivainen M. Enterovirus infection in human pancreatic islet cells, islet tropism in vivo and receptor involvement in cultured islet beta cells. Diabetologia 2004. 47:225-239. [DOD] [CrossRef]
- Tauriainen S, Oikarinen S, Oikarinen M, Hyoty H. Enteroviruses in the pathogenesis of type 1 diabetes. Semin Immunopathol 2011. 33:45-55. [DOD] [CrossRef]
- Hober D, Sane F, Jaidane H, Riedweg K, Goffard A, Desailloud R. Immunology in the clinic review series; focus on type 1 diabetes and viruses: role of antibodies enhancing the infection with Coxsackievirus-B in the pathogenesis of type 1 diabetes. Clin Exp Immunol 2012. 168:47-51. [DOD] [CrossRef]
- Marttila J, Juhela S, Vaarala O, Hyoty H, Roivainen M, Hinkkanen A, Vilja P, Simell O, Ilonen J. Responses of coxsackievirus B4-specific T-cell lines to 2C protein-characterization of epitopes with special reference to the GAD65 homology region. Virology 2001. 284:131-141. [DOD] [CrossRef]
- Green J, Casabonne D, Newton R. Coxsackie B virus serology and type 1 diabetes mellitus: a systematic review of published case-control studies. Diabet Med 2004. 21:507-514. [DOD] [CrossRef]
- Stene LC, Oikarinen S, Hyoty H, Barriga KJ, Norris JM, Klingensmith G, Hutton JC, Erlich HA, Eisenbarth GS, Rewers M. Enterovirus infection and progression from islet autoimmunity to type 1 diabetes: the Diabetes and Autoimmunity Study in the Young (DAISY). Diabetes 2010. 59:3174-3180. [DOD] [CrossRef]
- Tapia G, Cinek O, Rasmussen T, Witso E, Grinde B, Stene LC, Ronningen KS. Human enterovirus RNA in monthly fecal samples and islet autoimmunity in Norwegian children with high genetic risk for type 1 diabetes: the MIDIA study. Diabetes Care 2011. 34:151-155. [DOD] [CrossRef]
- Yeung WC, Rawlinson WD, Craig ME. Enterovirus infection and type 1 diabetes mellitus: systematic review and meta-analysis of observational molecular studies. BMJ 2011. 342:d35. [DOD] [CrossRef]
- Oikarinen S, Martiskainen M, Tauriainen S, Huhtala H, Ilonen J, Veijola R, Simell O, Knip M, Hyoty H. Enterovirus RNA in blood is linked to the development of type 1 diabetes. Diabetes 2011. 60:276-279. [DOD] [CrossRef]
- Lapinleimu K. A study of the occurrence of poliomyelitis and of neutralizing antibodies against the three types of poliomyelitis virus in Finland. Ann Med Exp Biol Fenn 1956. 34:1-104. [DOD]
- Oikarinen M, Tauriainen S, Oikarinen S, Honkanen T, Collin P, Rantala I, Maki M, Kaukinen K, Hyoty H. Type 1 diabetes is associated with enterovirus infection in gut mucosa. Diabetes 2012. 61:687-691. [DOD] [CrossRef]
- Dotta F, Censini S, van Halteren AG, Marselli L, Masini M, Dionisi S, Mosca F, Boggi U, Muda AO, Del Prato S, et al. Coxsackie B4 virus infection of beta cells and natural killer cell insulitis in recent-onset type 1 diabetic patients. Proc Natl Acad Sci USA 2007. 104:5115-5120. [DOD] [CrossRef]
- Wong KT, Munisamy B, Ong KC, Kojima H, Noriyo N, Chua KB, Ong BB, Nagashima K. The distribution of inflammation and virus in human enterovirus 71 encephalomyelitis suggests possible viral spread by neural pathways. J Neuropathol Exp Neurol 2008. 67:162-169. [DOD] [CrossRef]
- Khetsuriani N, Lamonte-Fowlkes A, Oberst S, Pallansch MA, Centers for Disease Control and Prevention. Enterovirus surveillance - United States, 1970-2005. MMWR Surveill Summ 2006. 55:1-20. [DOD]
- Sadeharju K, Knip M, Virtanen SM, Savilahti E, Tauriainen S, Koskela P, Akerblom HK, Hyoty H, Finnish TRIGR Study Group. Maternal antibodies in breast milk protect the child from enterovirus infections. Pediatrics 2007. 119:941-946. [DOD] [CrossRef]
- Smyth DJ, Cooper JD, Bailey R, Field S, Burren O, Smink LJ, Guja C, Ionescu-Tirgoviste C, Widmer B, Dunger DB, et al. A genome-wide association study of nonsynonymous SNPs identifies a type 1 diabetes locus in the interferon-induced helicase (IFIH1) region. Nat Genet 2006. 38:617-619. [DOD] [CrossRef]
- Shigemoto T, Kageyama M, Hirai R, Zheng J, Yoneyama M, Fujita T. Identification of loss of function mutations in human genes encoding RIG-I and MDA5: implications for resistance to type I diabetes. J Biol Chem 2009. 284:13348-13354. [DOD] [CrossRef]
- von Herrath M. Diabetes: A virus-gene collaboration. Nature 2009. 459:518-519. [DOD] [CrossRef]
- Viskari HR, Koskela P, Lonnrot M, Luonuansuu S, Reunanen A, Baer M, Hyoty H. Can enterovirus infections explain the increasing incidence of type 1 diabetes? Diabetes Care 2000. 23:414-416. [DOD]
- Viskari H, Ludvigsson J, Uibo R, Salur L, Marciulionyte D, Hermann R, Soltesz G, Fuchtenbusch M, Ziegler AG, Kondrashova A, et al. Relationship between the incidence of type 1 diabetes and enterovirus infections in different European populations: results from the EPIVIR project. J Med Virol 2004. 72:610-617. [DOD] [CrossRef]
- Viskari H, Ludvigsson J, Uibo R, Salur L, Marciulionyte D, Hermann R, Soltesz G, Fuchtenbusch M, Ziegler AG, Kondrashova A, et al. Relationship between the incidence of type 1 diabetes and maternal enterovirus antibodies: time trends and geographical variation. Diabetologia 2005. 48:1280-1287. [DOD] [CrossRef]
- Oikarinen S, Oikarinen M. Laboratory Diagnosis of Enterovirus Infection. In: Taylor K, Hyöty H, Toniolo A, Zuckerman AJ. Virus and diabetes. 2013, Sec. 13, pp. 118-119. [DOD]
- Parsonnet J, Friedman GD, Vandersteen DP, Chang Y, Vogelman JH, Orentreich N, Sibley RK. Helicobacter pylori infection and the risk of gastric carcinoma. N Engl J Med 1991. 325:1127-1131. [DOD] [CrossRef]
- An international association between Helicobacter pylori infection and gastric cancer. The EUROGAST Study Group. Lancet 1993. 341:1359-1362. [DOD] [CrossRef]
- Bosch FX, Lorincz A, Munoz N, Meijer CJ, Shah KV. The causal relation between human papillomavirus and cervical cancer. J Clin Pathol 2002. 55:244-265. [DOD] [CrossRef]
- Beasley RP. Hepatitis B virus. The major etiology of hepatocellular carcinoma. Cancer 1988. 61:1942-1956. [DOD] [CrossRef]
- Roivainen M, Ylipaasto P, Savolainen C, Galama J, Hovi T, Otonkoski T. Functional impairment and killing of human beta cells by enteroviruses: the capacity is shared by a wide range of serotypes, but the extent is a characteristic of individual virus strains. Diabetologia 2002. 45:693-702. [DOD] [CrossRef]
- Rother KI, Brown RJ, Morales MM, Wright E, Duan Z, Campbell C, Hardin DS, Popovic J, McEvoy RC, Harlan DM, et al. Effect of ingested interferon-alpha on beta-cell function in children with new-onset type 1 diabetes. Diabetes Care 2009. 32:1250-1255. [DOD] [CrossRef]
- Koivisto VA, Aro A, Cantell K, Haataja M, Huttunen J, Karonen SL, Mustajoki P, Pelkonen R, Seppala P. Remissions in newly diagnosed type 1 (insulin-dependent) diabetes: influence of interferon as an adjunct to insulin therapy. Diabetologia 1984. 27:193-197. [DOD]
- Henke A, Jarasch N, Wutzler P. Vaccination procedures against Coxsackievirus-induced heart disease. Expert Rev Vaccines 2003. 2:805-815. [DOD] [CrossRef]
- Gamble DR, Taylor KW, Cumming H. Coxsackie viruses and diabetes mellitus. Br Med J 1973. 4:260-262. [DOD] [CrossRef]
- Gamble DR, Kinsley ML, FitzGerald MG, Bolton R, Taylor KW. Viral antibodies in diabetes mellitus. Br Med J 1969. 3:627-630. [DOD] [CrossRef]
- Yoon JW, Austin M, Onodera T, Notkins AL. Isolation of a virus from the pancreas of a child with diabetic ketoacidosis. N Engl J Med 1979. 300:1173-1179. [DOD] [CrossRef]
- Jenson AB, Rosenberg HS, Notkins AL. Pancreatic islet-cell damage in children with fatal viral infections. Lancet 1980. 2:354-358. [DOD]
- Yoon JW, London WT, Curfman BL, Brown RL, Notkins AL. Coxsackie virus B4 produces transient diabetes in nonhuman primates. Diabetes 1986. 35:712-716. [DOD] [CrossRef]
- Laitinen OH, Honkanen H, Pakkanen O, Oikarinen S, Pulkki MM, Huhtala H, Ruokoranta T, Lecouturier V, Andre P, Harju R, et al. Coxsackievirus B1 induces beta-cell autoimmunity that portends type 1 diabetes. EUROPIC 2012 congress, 2012. St. Raphael, France. [DOD]
- Landau BJ, Whittier PS, Finkelstein SD, Alstein B, Grun JB, Schultz M, Crowell RL. Induction of heterotypic virus resistance in adult inbred mice immunized with a variant of Coxsackievirus B3. Microb Pathog 1990. 8:289-298. [DOD] [CrossRef]
- Oikarinen S, Tauriainen S, Huhtala H, Hober D, Lucas B, Gerasimidi-Vazeou A, Bozas E, Muir P, Honkanen H, Ilonen J, et al. Serological survey indicates association between Coxsackievirus B1 and type 1 diabetes in European populations – results from VIRDIAB study. 17th Europic meeting, 2012. Saint-Raphael, France. [DOD]
- Cabrera-Rode E, Sarmiento L, Tiberti C, Molina G, Barrios J, Hernandez D, Diaz-Horta O, Di Mario U. Type 1 diabetes islet associated antibodies in subjects infected by echovirus 16. Diabetologia 2003. 46:1348-1353. [DOD] [CrossRef]
- Davydova B, Harkonen T, Kaialainen S, Hovi T, Vaarala O, Roivainen M. Coxsackievirus immunization delays onset of diabetes in non-obese diabetic mice. J Med Virol 2003. 69:510-520. [DOD] [CrossRef]
- Graham S, Wang EC, Jenkins O, Borysiewicz LK. Analysis of the human T-cell response to picornaviruses: identification of T-cell epitopes close to B-cell epitopes in poliovirus. J Virol 1993. 67:1627-1637. [DOD]
- Seppala E, Viskari H, Hoppu S, Honkanen H, Huhtala H, Simell O, Ilonen J, Knip M, Hyoty H. Viral interference induced by live attenuated virus vaccine (OPV) can prevent otitis media. Vaccine 2011. 29:8615-8618. [DOD] [CrossRef]
This article has been cited by other articles:
|
Examining the gut bacteriome, virome, and mycobiome in glucose metabolism disorders: Are we on the right track?
Stefanakia C, Peppab M, Mastorakosc G, Chrousos GP
Metabolism 2017. 73:52-66
|
|
|
Viruses in type 1 diabetes
Hyöty H
Pediatr Diabetes 2016. 17(Suppl 22):56-64
|
|
|
Emerging Comorbidities in Adult Asthma: Risks, Clinical Associations, and Mechanisms
Kankaanranta H, Kauppi P, Tuomisto LE, Ilmarinen P
Mediators Inflamm 2016. 2016:3690628
|
|
|
Examination of a Viral Infection Mimetic Model in Human iPS Cell-Derived Insulin-Producing Cells and the Anti-Apoptotic Effect of GLP-1 Analogue
Baden MY, Fukui K, Hosokawa Y, Iwahashi H, Imagawa A, Shimomura I
Plos One 2015. 10(12):e0144606
|
|
|
Influenza A virus antibodies show no association with pancreatic islet autoantibodies in children genetically predisposed to type 1 diabetes
Kondrashova A, Nurminen N, Patrikainen M, Huhtala H, Lehtonen J, Toppari J, Ilonen J, Simell OG, Veijola R, Knip M, Hyöty H
Diabetologia 2015. 58(11):2592-2595
|
|
|
Developing a vaccine for Type 1 diabetes through targeting enteroviral infections
Hyöty H, Knip M
Expert Rev Vaccines 2014. 13(8):989-999
|
|
|
Virus antibody survey in different European populations indicates risk association between coxsackievirus B1 and type 1 diabetes
Oikarinen S, Tauriainen S, Hober D, Lucas B, Vazeou A, Sioofy-Khojine A, Bozas E, Muir P, Honkanen H, Ilonen J, Knip M, Keskinen P, Saha MT, Huhtala H, Stanway G, Bartsocas C, Ludvigsson J, Taylor K, Hyöty H, VirDiab Study Group
Diabetes 2014. 63(2):655-662
|
|
|